Often possessing more than one binding site, lectins are thus intrinsically multivalent receptors that can interact with multiple copies of a monovalent ligand at once. For instance, a high display of carbohydrate epitopes is represented by the glycocalyx, which is the complex carbohydrate coating that decorates the cellular surface. The glycocalyx is an illustration of how a large number of carbohydrate epitopes organize and are available for multiple interactions. By introducing more binding partners in this recognition event, the complexity of the overall interaction is higher. Some mechanisms that influence the final affinity (named avidity in multivalency nomenclature) can potentially occur at the same time. They happen via an inter- or intra-molecular fashion or a combination of the two. The following terms, such as chelation, statistical rebinding, receptor clustering and aggregation, define these mechanisms (Fig 3).
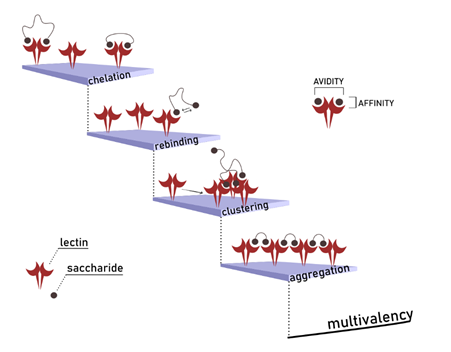
Since its first appearance in literature as “cluster glycoside effect”, (Lundquist & Toon, 2002; Lee & Lee, 1995; Mammen et al., 1983) a lot of effort have been devoted to the analysis and quantification of the enhancement in affinity. The evaluation of the binding constants of multivalent binding is not always so straightforward. A range of assays is now available to evaluate the binding of n carbohydrate ligands in m lectin binging sites (the same are chosen when n = 1 for a monovalent interaction). The commonly used ITC (isothermal titration calorimetry) and SPR (surface plasmon resonance) constitute two of the most potent methods.
The phenomenon of multivalency, as detected from different techniques, appears more complex than the simple sum of monovalent affinities. Instead, observed potencies are amplified from individual weak carbohydrate-protein interactions without a fixed proportion. Binding sites on the lectin surface are independent one from each other, which makes multivalency not a cooperative event in the traditional sense of the term. The β value is used to quantify the benefit resulted from such sort of interactions, (Reynolds & Perez, 2011) instead of the more familiar α parameter, generally calculated in cooperativity (for example in the classic case of oxygen binding to haemoglobin). As such β value is an affinity enhancement factor ; it is defined as the ratio of the global equilibrium constant to the monomeric equilibrium association constant. (Mammen et al., 1998)
The obstacle in interpreting the observed data is the difficulty to determine the effect of the interplay of many concomitant “micro” mechanisms of interaction to the macroscopic affinity. Nevertheless, the thermodynamics of multivalent glycoside-lectin interaction has been investigated extensively (Mammen et al., 1998; Reynolds & Perez, 2011; Gomez-Casado; 2011; Curk et al., 2017; Kitov & Bundle, 2003). This knowledge has been utilised to rationalise observations on biological events of medicinal relevance, to evaluate the potency of glyco-drugs as lectin inhibitors or to exploit avidity in the construction of more and more responsive biosensors. The determination of Kd or IC50 values for a glycoside to its targeted lectin is of fundamental importance for these types of applications. Such measurements are usually simple when the ligand-lectin interaction is monovalent. In contrast, the evaluation of a multivalent association is often complicated by the “hostile” interpretation of the results, solubility/aggregation issues or merely a limited access to appropriate instrumentation. However, some convincing solutions could be found within a given set of assumptions and conditions.
To explore the effect of exclusive chelation, we will consider protein bridging by a divalent glycoside. Under these circumstances, the whole complexation process can be analysed stepwise. The first event is the binding of one of the two ligands in one protein pocket. The following binding interaction occurs after the first. At this moment, the lectin experiences a higher effective concentration of the ligand on its surface, since the second monomer can be found already nearby of a binding site, vicinal to the one already occupied. The increased density of local concentration results in lower Kd for the overall binding event. The range of this improvement is dependent on linker flexibility and length since these features dictate the probability of the intramolecular event to occur. Affinity enhancements for a divalent sialyl-LacNAc conjugate were modelled in the group of Seitz (Bandlow, et al., 2017) and used to establish a distance-activity relationship for the interaction with trimeric Influenza A virus hemagglutinin. The same assumption for a rod-like ligand was adopted to predict Kd of a divalent LecA inhibitor and compared with the experimental data. (Yu et al., 2019)
Avidity can also be studied as the result of an intermolecular process. The accumulated binding strength derives from multiple affinities experienced by high-valence glycosides in recruiting lectins (receptor clustering) and binding more at the same time (cross-linking). Strategies that involve multivalent intermolecular interactions dominate a myriad of biological events. They generally are associated with the rise of local receptor/glycoconjugate concentration. The result is an increase in the strength of binding or facilitation of the diffusion of a polyvalent partner trough the cellular membrane. The well-known attachment of influenza virus to host tissue via HA-SA (hemagglutinin-sialic acid) (Mammen et al., 1995) is an example of such natural processes. (Fig. 4)
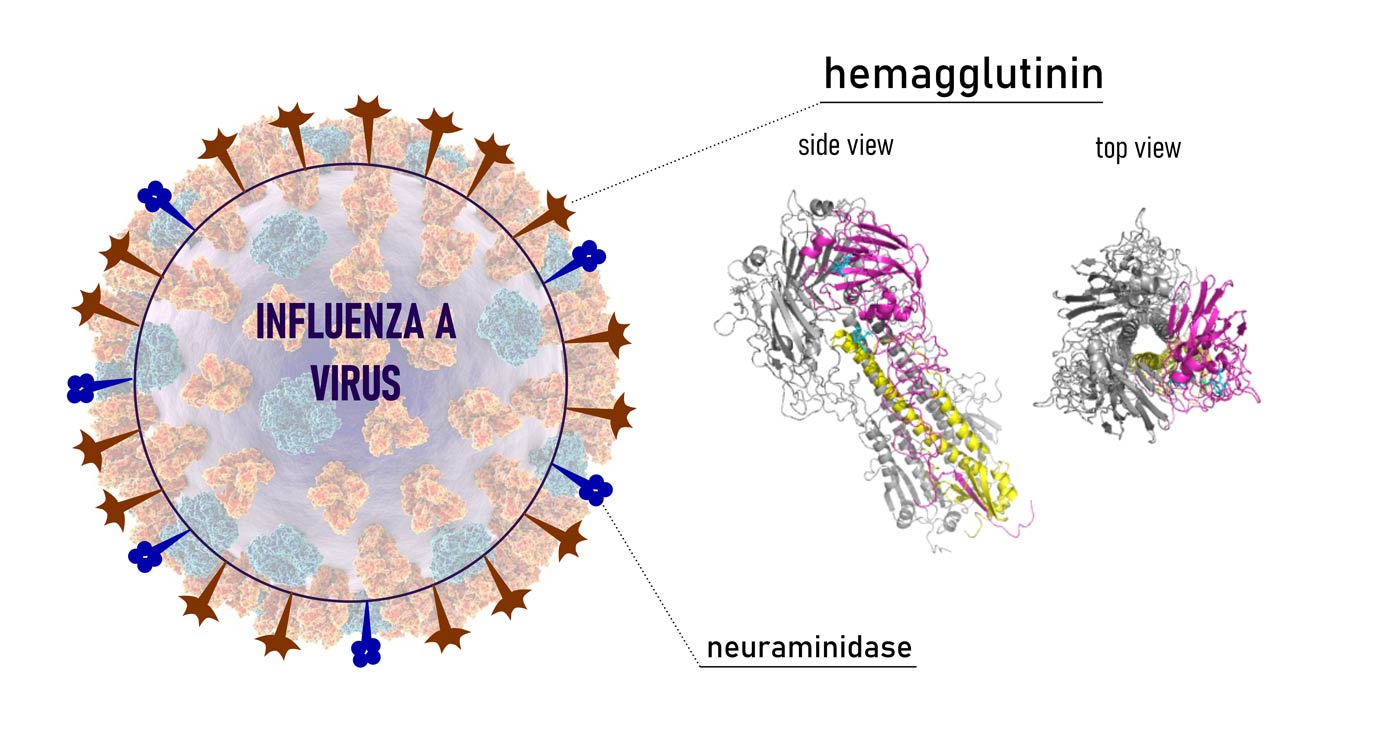
An event that again relies on simultaneous and specific contacts between multimeric binding partners is the aggregation of lectins by cell-surface mucins, known to induce signal transduction effects such as apoptosis or regulation of the T-cell receptor. (Dam & Brewer, 2010}
Studies on the binding thermodynamics of multivalent mannosides to legume lectins (concavallin A (ConA) and Dioclea grandiflora lectin (DGL)), that have binding sites too far apart to be spanned by a single glycosylated molecule, were reported by Dam and Brewer (Dam et al., 2002). Their results indicated that the multi-mannosides bind to the lectin with decreasing affinity, which can be partially explained by the progressive decrease of functional valence as they bind. Similar studies on soybean agglutinin (SBA) binding to porcine submaxillary mucin (PSM)(Dam et al., 2009) report negative cooperativity and a decrease in entropy of binding after highly favourable initial TΔS. The authors described this behaviour as “bind and jump”, a dynamic binding process distinct from the classical ‘‘lock and key’’ model. SBA moves along the mucin from one sugar residue to another, progressively reducing diffusion as cross-linking takes place and restricts the movement of the lectin.
Avidity often leads to the formation of non-covalent complexes in which a multivalent glycan aggregates several lectin molecules in the form of chains or networks. This behaviour can lead to both soluble and insoluble complexes. The ability to cross-link lectins is essential for receptor clustering in biology since a lot of signalling and recognition processes depend on it. Various publications have shown how cluster formation is influenced by many parameters, such as the valency, spacing and flexibility of the carbohydrate epitopes (Gestwicki et al., 2005; Dam et al., 2005; Boukareb et al., 2014). The geometry of these aggregates can be revealed by atomic force microscopy. This technique was used by Sicard and co-workers (Sicard et al., 2011) to visualise the cluster architecture of a tetra-galactosylated calix[4]arene with LecA lectin from Pseudomonas aeruginosa. Interestingly, the strength of multi-galactosyl-lectin interaction depends on the topology and valence of the glycol-cluster itself.
Despite these successful examples, our understanding of multivalency is still limited. In the last 20 years, more attention was put in the development of design strategies for small molecules and glycomimetics (Ernst & Magnani, 2009) than in the study of multivalent systems on the molecular level, even though they are ubiquitous in biology. Recently, new concepts started to appear and to fill gaps in the theoretical understanding of the phenomenon of multivalency. The idea of “super selectivity”, (Curk et al., 2017) for example, was developed to explain the switch-like behaviour that is typical with many regulatory processes and usually occurs in an environment in which the receptor is in excess. Super-selective behaviour can be generated by weak multivalent interactions whose strength increases as the density of the receptors also grows (and therefore, the number of possible bonds raises too).
One of the limits for the design of multivalent scaffolds for pharmaceutical purposes results from the fact that many parameters contribute to the multivalent interaction (Bhatia et al., 2016). A fine-tuning of the synthetic candidate has to calibrate valency, size, flexibility and extent of hydration of the glycoside, but also take into account receptor concentration and its solvation shell. Sometimes these features contribute to the multivalency effect in a counter-intuitive fashion. The highest valency is not always the requisite for the strongest interaction. Also, lower concentration of the multivalent ligand and a weak initial binding generally are beneficial in terms of selectivity (Lanfranco et al., 2019). A lack of tools to assay polyvalent protein-carbohydrate interactions may prevent the effective development of multivalent drugs.
Methods available require further development to address the questions related to the role of glycans in biology, as well as the evaluation of new glyco-drugs as lectin inhibitors. In the next paragraphs, we will give an overview of the most extensively used techniques for these purposes. A critical guide will accompany them on the interpretation of the results, which is fundamental for the multivalent case even more than it is for monovalent one. We hope this guide will assist the selection of the most appropriate detection methods and how to use them.