The greatest problem associated with NOE experiments is the zero-crossing region around ω0τc ≈ 1 where the conventional (laboratory-frame) NOE observed via steady-state or transient techniques becomes vanishingly small. This typically occurs for mid-sized molecules with masses of around 1000-2000 Da, depending on solution conditions and spectrometer frequency. With the increasing interest in larger molecules in many areas of organic chemistry research coupled with the wider availability of higher field instruments, this is likely to be a region visited ever more frequently by the research chemists’ molecules. Other than altering solution conditions (such as changing temperature) in an attempt to escape from this, the measurement of NOEs in the rotating-frame provides an alternative solution. In this case, the cross-relaxation rate between homonuclear spins is given by an expression that remains positive for all values of τc, and the undeniable benefit of ROEs is, quite simply, that they remain positive for all realistic molecular tumbling rates. For small molecules, the magnitude of the ROE matches that of the transient NOE, whilst for larger molecules it reaches a maximum for homonuclear spins of 68%, but under no circumstances does it become zero (Fig.2 2). Similarly, the NOE and ROE growth rates are identical for small molecules but differ for very large ones.
For very large molecules, the ROE therefore grows twice as fast as the NOE, and has opposite sign (Farmer et al., 1969). In essence, ROEs develop whilst magnetisation is held static in the transverse plane, rather than along the longitudinal axis (hence they are sometimes also referred to as transverse NOEs). To generate the required population disturbance of the source spins, the target resonance is subjected to a selective 180º pulse prior to the non-selective 90º pulse, such that it experiences a net 270º flip and is thus inverted relative to all others. Transverse magnetisation is then “frozen” in the rotating frame by the application of a continuous, low power spin-lock pulse to prevent evolution (in the rotating frame) of chemical shifts. The experiment is more frequently performed as the 2D experiment where it is usually termed ROESY (rotating-frame NOE spectroscopy). The situation during the spin-lock may be viewed as the transverse equivalent of events during the transient NOE mixing time (Fig. 3). The action of the spin-lock is to maintain the opposing disposition of magnetisation vectors, which would otherwise be lost through differential chemical shift evolution, and so allows the ROE to develop through cross-relaxation in the transverse plane. Spin relaxation here is characterised by a time constant called T1ρ, of very similar magnitude to T2. In utilising the spin-lock, one has effectively replaced the static BO field of the conventional NOE with the far smaller rf B1 field, and it is this that changes the dynamics of the NOE. Whereas γB0 typically corresponds to frequencies of hundreds of megahertz, γB1 is typically only a few kilohertz, meaning γB1 << γB0 and hence ω1 (the rotating-frame frequencies) << ω0. The consequence of this is that ω1τc << 1 for all realistic values of τc, and all molecules behave as if they are within the extreme narrowing limit. Thus, ROEs are positive, any indirect effects have opposite sign to direct effects and tend to be weak, and saturation transfer can be distinguished by sign from ROEs, regardless of molecular size and dynamics. Against these obvious benefits are a number of experimental problems, principally TOCSY transfers (particularly contributing to strongly coupled systems such as glycans), also occurring during the spin-lock, and signal attenuation from off-resonance effects.
An alternative ROESY sequence is the T-ROESY experiment, which is effective at suppressing TOCSY transfer. This is achieved by substituting the low-power continuous wave lock field used in ROESY sequence by a mixing sequence of 180ºx 180º-x or 180ºx 180º-x 360ºx 360º-x 180ºx 180º-x pulses. However, cross-relaxation rates in T-ROESY are an equal mixture of ROE and NOE components, and moreover, the cross-relaxation rates measured are four times lower than those in conventional ROESY (Fig. 2) (Ravikumar et al., 1993).
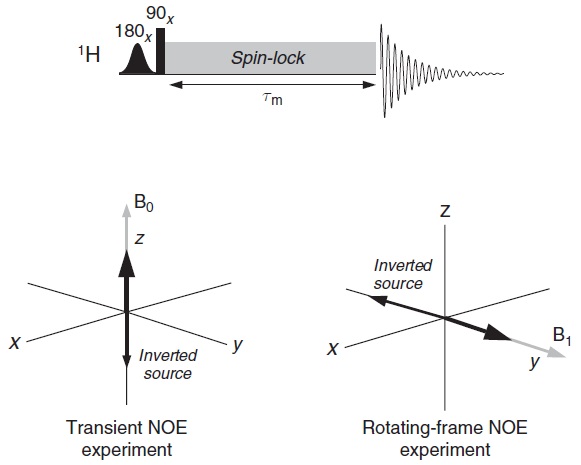
Figure 3. (Up) Scheme of the pulse sequence for observing rotating-frame NOEs. The ROEs develops during the long spin-lock pulse that constitutes the mixing period τm. (Down) Situation of the magnetization during the spin-lock. The rotating-frame NOE experiment can be viewed as the transverse equivalent of the transient NOE experiment.