4.1. Extraction of Chitin
The primary commercial sources of chitin are crab and shrimp shells. The isolation of chitin begins with the selection of shells, which has an important bearing on the subsequent quality and property of the final isolated material. For example, for lobsters and crabs, shells of the same size and species are chosen. In the case of shrimps, where the cell wall is thinner, the chitin isolation is easier than from other types of shells. The selected shells are then cleaned, dried and ground into small shell pieces. In industrial processing, chitin is extracted by acid treatment to dissolve the calcium carbonate followed by an alkaline solution to dissolve proteins. Also, a decolorization step is often added to remove pigments and obtain colorless pure chitin. All those treatments must be adapted to chitin source, owing to differences in the ultrastructure of the original material, to produce first high-quality chitin, and then chitosan (after partial deacetylation). Chitin is infusible and sparingly soluble during the transformation into different conformations. The question of its solubility is a major problem in the development of both processing and use of chitin as well as its characterization.
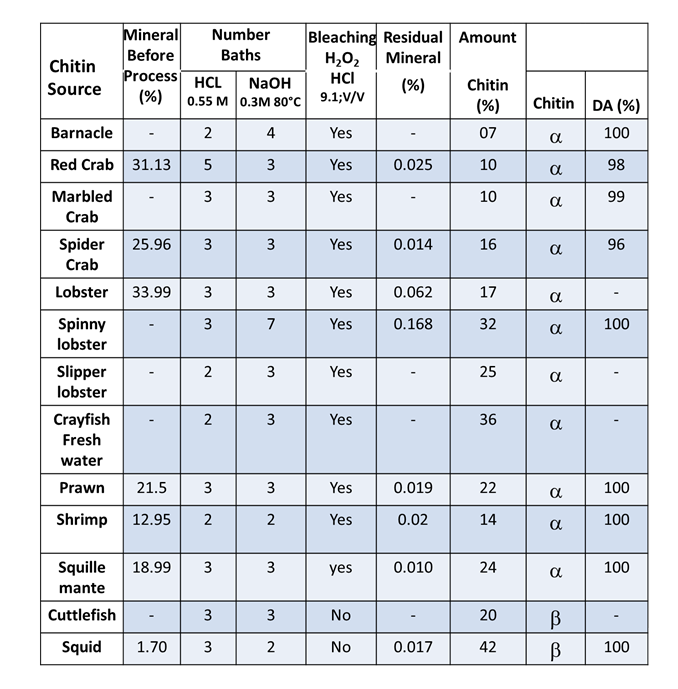
4.1.1. Chemical extraction
4.1.1.1. Chemical demineralization. The process consists in the removal of minerals, primarily calcium carbonate, using hydrochloric acid as a preferred reagent. Demineralization is easily achieved because it involves the decomposition of calcium carbonate into the water-soluble calcium salts with the release of carbon dioxide. Most of the other minerals present in the shell-fish cuticle react similarly and give soluble salts in the presence of acid. Then, salts can be separated by filtration of the chitin solid-phase followed by washing using deionized water.
4.1.1.2. Chemical deproteinization. The deproteinization step requires the disruption of the chitin protein-complex and the solubilization of proteins. Such a step is performed heterogeneously using chemicals that also depolymerize the biopolymer; the results depend from the experimental conditions used. The complete removal of protein is especially essential for biomedical applications, as a percentage of the human population is allergic to shellfish, the primary culprit being the protein component.
The processes of chemical deproteinization and demineralization affect the molecular weights and degree of acetylation of chitin. The use of solid-state NMR provides a way to assess the degree of acetylation. The higher the degree of acetylation, the less degradation has occurred to the native polymer sample. The use of successive baths of lower HCl concentrations (0.55M) and NaOH (0.3 M) concentrations preserves the native chitin as shown from the results obtained for 12 different species of crustaceous and cephalopods for which the degree of acetylation was varying between 96% and 100%. (Tolaimaite et al., 2008)
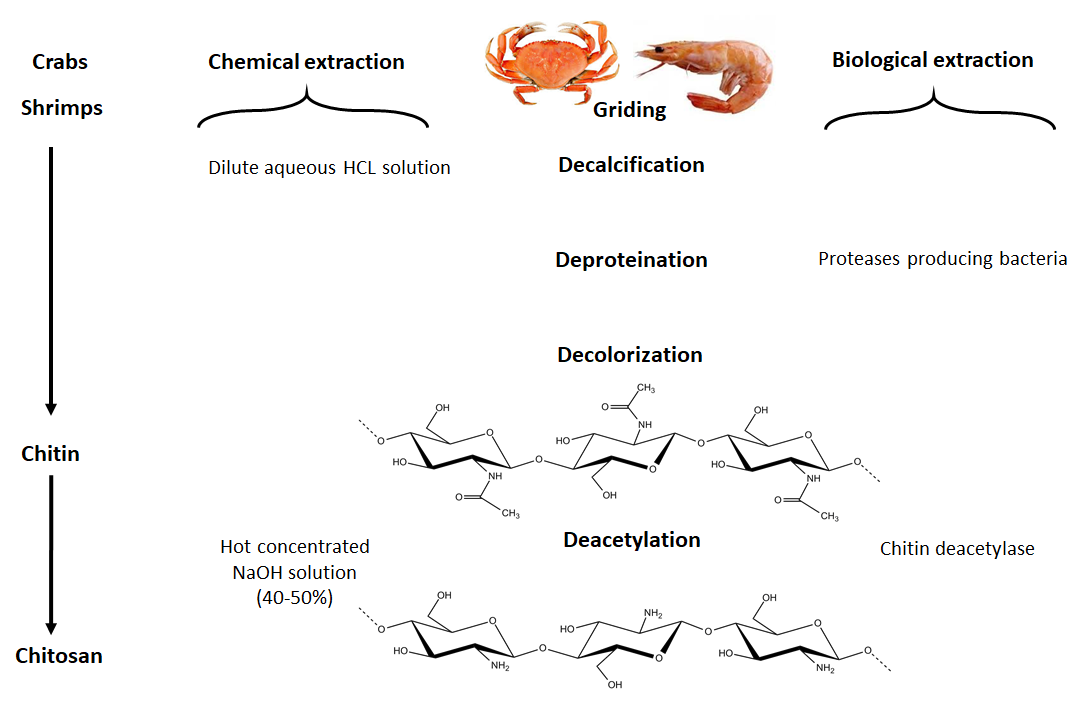
The chitin and chitosan chemical and biological processes.
4.1.2. Biological extraction
The use of enzymes in the deproteinization step appears as early as in 1934, which lead to the foundations and the developments of the fermentation process. Comparative studies between chemical and biological processes for extracting chitin from shrimp’s shells point toward a preference for the uses of enzymes and microorganisms for chitin extraction. While offering several advantages such as simplicity, producibility, low solvent, and energy consumption, the process yields the chitin of high molecular weight. The fermentation is achieved by a process called auto-fermentation, by endogenous microorganisms or by adding selected strains of microorganisms. Fermentation methods follow two different pathways; namely lactic acid fermentation and non-lactic acid fermentation. The lactic acid fermentation requires to inoculate the well ground shell-fish waste with a lactic acid culture and a carbohydrate source while being mixed thoroughly. The production of lactic acid decreases the pH, thereby dissolving the CaCO3, and concomitant proteolysis by the enzymes from the shellfish viscera. (Younes & Rinaudo, 2015)
A somehow related approach to this, in which the deproteinization and demineralization steps are separated, requires the action of both bacteria (Bacillus strains) and fungi. Many processes and substrates have been investigated. One of this used Aspergillus niger, taking advantage of the action of the enzymes released by the fungi for their deproteinization and partial demineralization of crustacean shells. Many factors influence the fermentation process and the subsequent efficiency of deproteinization and demineralization. The biotechnological process must be completed by further mild chemical treatment to remove the extra protein and minerals and yield highly purified chitin.
4.2. Chitosan preparation
The term usually refers to a family of polymers obtained after chitin partial deacetylation to varying acetylation degrees (DA). The acetylation degree, which reflects the balance between the two types of residues differentiates chitin from chitosan. All (1-4)-linked copolymers of β-D-GlcNAc and β-D-GlcN units should be designated as chitin or chitosan-based on their insolubility or solubility, respectively. During deacetylation, acetyl groups are removed randomly or non-random but also depolymerization reaction occurs, indicated by changes in MW of chitosan. Several factors influence the structures and properties of chitosan. These are the degree of polymerization (DP), the heterogeneity of the molecular weight, the fraction of acetylation, and the pattern of acetylation.
Although chitosan occurs naturally in some fungi and green algae, it is primarily produced industrially from chitin by chemical treatment using alkali. These methods are used extensively for commercial purpose of chitosan preparation because of their low cost and suitability to mass production. From a chemical point of view, alkalis can be used to deacetylate chitin. Commonly, in the heterogeneous method, chitin is treated with a hot concentrated solution of NaOH during a few hours, and deacetylated up to ∼85%–99% and chitosan are produced. According to the homogeneous method, alkali chitin is prepared after the dispersion of chitin in concentrated NaOH (30 g NaOH/45 g H2O/ 3 g Chitin) at 25 °C for three hours or more, followed by dissolution in crushed ice around 0 °C. This method results in soluble chitosan with an average degree of acetylation of 48%–55%. Many parameters in the deacetylation reaction can limit the characteristics of the final chitosan. The results shown in Table 2, show the advantage of successive bathes compared to a single treatment of a long time ; the importance of the addition of NaBH4 preventing oxidation is clearly demonstrated.
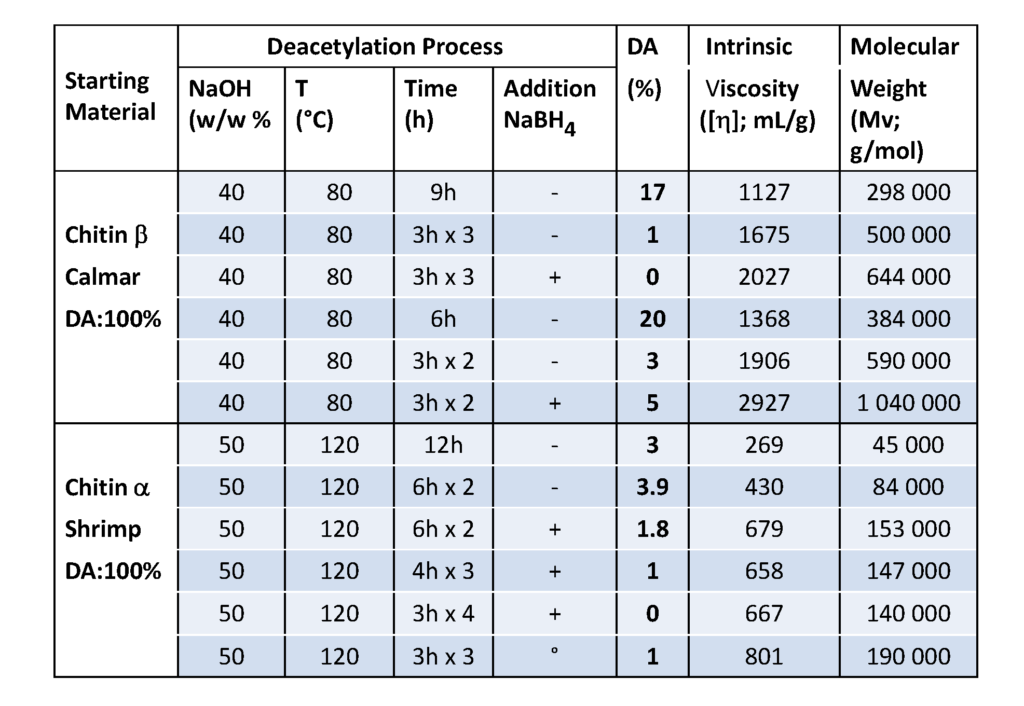
Chitin is converted to chitosan by enzymatic preparations. Such methods, exploiting chitin deacetylases offer the possibility of a controlled non-degradable process that yields well-defined chitosans, both in size, degree of acetylation, and pattern of acetylation. Chitin deacetylase (EC 3.5.1.41) catalyzes the hydrolysis of N-acetamido bonds in chitin to produce chitosan. Since chitin is hard to break due to its physicochemical properties, its degradation usually requires the action of more than one enzyme type. In several instances, pretreatment of chitin substrates before enzyme addition is required to improve the accessibility of the acetyl groups to the enzyme and therefore to enhance the deacetylation yield.
Endo-splitting chitinases produce chitooligomers that are subsequently converted to monomers by exo-splitting β-N-acetylglucosaminidases. The latter enzyme cleaves off N-acetylglucosamine units from non-reducing ends and prefers smaller substrates than chitinases. (Koga et al., 1982, 1983, 1997; Fukamizo and Kramer, 1985a, b; Kramer and Koga, 1986); Kramer et al., 1993; Zen et al., 1996; Filho et al., 2002 As a consequence, of these properties, the overall rate of chitin hydrolysis is limited by the action of the chito-oligomer-producing chitinase, which drastically increases.