As previously mentioned, multidrug-resistant (MDR) pathogens are a constant threat to hospitalized patients, especially those with risk factors such as cancer, diabetes, immunodeficiency, etc. Indeed, opportunistic pathogens take advantage of their weakened organisms for infecting and spreading among patients, leading to outbreaks of hospital-acquired infections (HAIs). Among MDR opportunistic pathogens, lung pathogens are especially notorious: lower respiratory infections have been the fourth leading cause of death for the last 20 years. (WHO, 2020) Among the victims of lower respiratory infections, cystic fibrosis patients are particularly vulnerable: in their case, infection by an MDR lung pathogen can easily translate into a death sentence.
Indeed, lung pathogens thrive in the conditions created by CF: thick mucus hinders the action of immune factors and therapeutic agents and reduces the ability for airway cleansing through mucociliary clearance or expectoration. Infected patients often carry infections by one or multiple pathogens throughout the years. Even the most invasive interventions such as lung transplantation do not guarantee recovery. What is more: re-infection of the lungs after transplant is not uncommon, meaning that the confirmed presence of lung pathogens can be a decisive factor leading to a denial of this life-saving procedure.(Ciofu et al., 2013; O’Sullivan et al., 2009) The list of pathogens associated with chronic lung infection is long: Pseudomonas aeruginosa, Staphylococcus aureus, Haemophilus influenza, Achromobacter xylosoxidans, Stenotrophomonas maltophilia, and members of the genus Burkholderia, with more being discovered over time. (Ciofu et al., 2013) Two notorious specimens are P. aeruginosa and B. cenocepacia, albeit for different reasons (Figure 9).
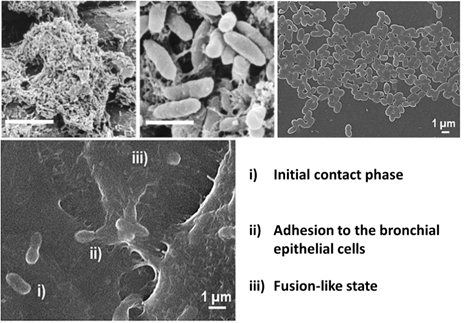
P. aeruginosa forms part of the ESKAPE pathogens: high-profile threats to human healthcare. In 2017, it was set as a priority for developing new antibiotics by the WHO.(WHO, 2017) P. aeruginosa is a Gram-negative bacterium that displays intrinsic drug resistance and quickly develops MDR in clinical settings. It is not an inherently easy target to treat, yet P. aeruginosa lung infections rarely affect healthy individuals. It is due to its opportunistic behaviour: in humans, infections by this pathogen occur in conjunction with ailments such as eye injuries, burns, immunodeficiency (AIDS, cancer), and, above all, inflammatory airway diseases (CF, asthma, chronic obstructive pulmonary disease). (Diggle et al., 2020; Garcia-Clemente et al., 2020) P. aeruginosa is responsible for many HAIs (10 % worldwide) and is mainly responsible for mortality in CF populations. Two factors are responsible for its high impact: its ubiquitous presence and its capacity to form biofilms. Firstly, this bacterium finds its way to hospitals by virtually every possible path: newly admitted visitors and patients, unsterilized medical equipment, aerators, water supply, and even healthcare personnel. (van Duin et al., 2016) Secondly, once it establishes itself in a host organism, this pathogen can deploy biofilm and even change its phenotype from ‘non-mucoid’ to ‘mucoid’, meaning that it becomes increasingly persistent in antibiotic treatment and deploys factors to boost its virulence. (Diggle et al., 2020; Garcia-Clemente et al., 2020) This adaptive plasticity is a testimony of how difficult is to eradicate infections by P. aeruginosa permanently.
Among its many virulence factors, P. aeruginosa produces two widely studied lectins: LecA and LecB, formerly known as PA-IL and PA-IIL. (Gilboa-Garber, 1982) Regulated by quorum sensing, these lectins are released into the extracellular matrix and are known to be essential to biofilm formation, meaning they play a vital role in the infection bio-machinery.(Diggle et al., 2006; Passos da Silva et al., 2019; Tielker et al., 2005; Winzer et al., 2000) Moreover, both have shown similar roles in pathogenicity either by mediating cell adhesion, blocking epithelial ciliary beating or having a cytotoxic effect on lung cells.(Adam et al., 1997; Bajolet-Laudinat et al., 1994; Chemani et al., 2009)
As such, both lectins have become targets for AAT: in vitro and in vivo studies demonstrated the usefulness of using the corresponding sugars (galactose, mannose, fucose) for inhibiting the effects of LecA and LecB.(Bucior et al., 2013; Chemani et al., 2009; Diggle et al., 2006) A small pilot study explored the treatment of CF patients by inhalation of monosaccharides: the treatment was well-tolerated and led to promising results, but the size of the study limited any claims. (Hauber et al., 2008) Further down the line, mono- and multivalent glycomimetics were developed, with ever-improving affinities and inhibition effects.(Boukerb et al., 2014; Gustke et al., 2012; Sommer et al., 2019; Sommer et al., 2018) Moreover, as prototypical targets for AAT, these lectins have been used to test innovative strategies such glycomimetic-mediated antibiotic delivery and the first case of covalent lectin inhibition. (Meiers et al., 2020; Wagner et al., 2017) These recent advancements on the glycomimetic avenue, along with advances in many other fields, hold promise in treating P. aeruginosa infections. Indeed, decades of study and efforts may remove P. aeruginosa from its place among the most threatening lung pathogens in the not-so-distant future.
The information thus presented concerning P. aeruginosa can also be related to the main lung pathogen described in this text: Burkholderia cenocepacia. In many aspects, B. cenocepacia bears a close resemblance to P. aeruginosa: it is opportunistic, multidrug-resistant, ubiquitous in the environment, and has led to HAIs in the same way P. aeruginosa has. (Tavares et al., 2020) Furthermore, B. cenocepacia mediates its infection through quorum sensing, adhesion and virulence vectors.(Loutet et al., 2010; Mil-Homens et al., 2012; Pimenta et al., 2021; Scoffone et al., 2017) Lastly, it has shown the ability to modulate its phenotype during chronic infection and form biofilms, even in cooperation with P. aeruginosa.(Bragonzi et al., 2012; Inhulsen et al., 2012; Mira et al., 2011) On the other hand, key differences exist between these high-profile pathogens: for instance, B. cenocepacia also infects patients suffering from a chronic granulomatous disease (CGD). (Speert et al., 1994) More importantly, its drug-resistance profile is broader, and its lung infections are much more likely to spread than P. aeruginosa.(Campana et al., 2005; Mahenthiralingam et al., 2005; van Duin et al., 2016) Adding to this, although B. cenocepacia affects fewer patients than P. aeruginosa, its pathogenicity is much more severe and associated with worse patient outcomes. Its infections are usually considered more concerning than those of P. aeruginosa. (Tavares et al., 2020)
Indeed, B. cenocepacia, along with more than 20 strains of the Burkholderia genus, have been compiled in the BCC: Burkholderia cepacia complex. (Vandamme et al., 1997) The BCC was introduced in 1997 by the ‘International Burkholderia cepacia Working Group’ – IBCWG, which was assembled to discuss the emerging threat to public health. (IBCWG; Vandamme et al., 1997) Among the BCC species, a handful is defined by their role as opportunistic pathogens in lung infection: infection outcomes range from asymptomatic carriage to chronic infection and, in the worst cases, deadly ‘cepacia syndrome’. Cepacia syndrome defines a rapid exacerbation of the pulmonary infection: necrotizing pneumonia and septicaemia lead to systemic infection and, if left untreated, death.(Mahenthiralingam et al., 2005) Although sometimes cured, this syndrome is considered almost untreatable. Due to this, CF patients infected with B. cenocepacia and other BCC members are often segregated to protect other susceptible patients. (Jones et al., 2004)
In recent times BCC bacteria have sparked severe predicaments: year-spanning outbreaks of B. stabilis in Swiss hospitals were studied in 2019, tracing the origin of the contamination to commercially available gloves. (Seth-Smith et al., 2019) Similarly, in 2019, the French ANSM (National Agency for Medicines and Health Products Safety) had to release an alert recalling batches of contaminated disinfectant agents swiftly. (2019; ANSM, 2019) It happened after the manufacturer Anios, a European market leader in terms of hospital-related disinfection, reported two of their products were contaminated by bacteria: B. cepacia and Pseudomonas oryzihabitans. (Carlotti, 2020; Duthoit, 2019) In this case, the origin of the bacteria was traced to the water supply, highlighting the fact that these ubiquitous bacteria represent a pervasive threat.
Among the species in the BCC, B. cenocepacia is multidrug-resistant and is the species most commonly transmitted among BCC-infected populations and often accounts for half or more of the total BCC-infections among the studied CF populations.(Campana et al., 2005; Drevinek et al., 2010; Scoffone et al., 2017) Lastly, B. cenocepacia is responsible for cepacia syndrome, making it the deadliest species from its genus. It is undoubtedly related to its prevalence but is also a testament to the particularly aggressive infections that B. cenocepacia elicits compared to other members of the BCC. (Jones et al., 2004) In conjunction, these facts explain why infection with B. cenocepacia is considered most critical and has been studied the most.
Before its reclassification to ‘cenocepacia’ in 2003, the pathogenic traits of this species were observed early on as B. cepacia’s genomovar III. (Vandamme et al., 2003; Vandamme et al., 1997) Extensive study of this bacterium has continued today, from its genome sequencing in 2009 to recent studies dissecting virulence, pathogenicity, existing treatments, and new possible therapies. (Holden et al., 2009; Loutet et al., 2010; Pimenta et al., 2021; Regan et al., 2019; Scoffone et al., 2017) The current stance in terms of treatment remains antibiotic combination therapy: early aggressive treatment may prevent chronic BCC infections. Nevertheless, in the particular case of B. cenocepacia, infections become chronic in over 90% of the cases. (Regan et al., 2019) Because no consensus on a standardized protocol for treatment exists, the recurring conclusion is that better tools are needed to understand and treat infections by B. cenocepacia.
On a different note, pioneering work on gene editing has shown that B. cenocepacia and one of its toxins may hold the key to accomplishing mitochondrial gene editing. (Mok et al., 2020) This discovery highlights the importance of exploring a pathogenic target through all the available avenues, which may uncover therapeutic potential or other unexpected applications. As stated previously, recent review articles have explored B. cenocepacia and its machinery in terms of determinants for biofilm formation and quorum sensing, adhesins, toxins, etc. Nevertheless, those studies seem to have overlooked the existence of soluble lectins in the proteome of B. cenocepacia.