In order to act efficiently, infective pathogens need to interact with their environment. First and foremost, a virus or a bacterium needs to recognize the cells of its host to start the infective process. At this point, it becomes necessary for the pathogen to remain in close vicinity to its host cells. In this vicinity, pathogens thrive: enhanced access to nutrients, shelter from cleansing mechanisms such as airflow or liquid flow, cover from immune factors all converge to facilitate infection. Consequently, host-adhesion is a determinant factor in the infective process.
The concept of anti-adhesion therapy surfaced in the 90s and consisted of using monoclonal antibodies to disrupt adhesive interactions between leukocytes and endothelial cells. Disrupting those interactions was therapeutically beneficial in models of inflammation or immune response. (Carlos et al., 1994) Currently, AAT still aims to disrupt adhesive interactions but has broadened its scope considerably. One of its main applications is relevant to our study: to disrupt the interactions between invasive pathogens and their hosts (see Figure 1).
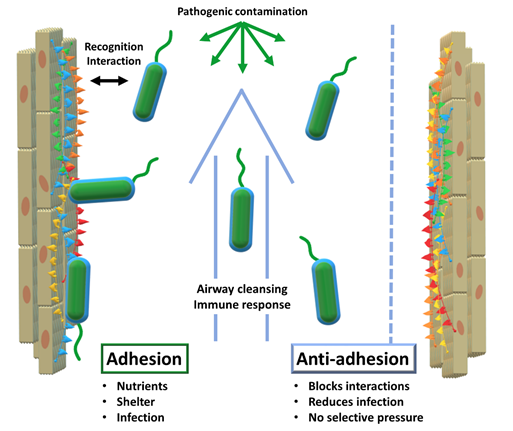
Considering the increasingly complex challenges to antibiotic therapy and the emergence of drug-resistant fungal pathogens, anti-adhesion has gained momentum as a complementary type of therapy. AAT can be deemed complementary to antibiotic therapy because of its lack of selective evolutionary pressure: with antibiotics, only the drug-resistant mutants survive and constitute the next generation. Conversely, AAT does not result in the elimination of pathogens. By merely obstructing the infective action of the pathogens, this type of therapy does not induce selective pressure in such a direct way. Nevertheless, it is arguable that mutant organisms that evade AAT and proceed to successful infection will gain an evolutionary advantage: enhanced access to nutrients and capacity to multiply, especially for viruses. Alternatively, these ‘favoured’ strains will have to compete with normal strains, instead of being the sole survivors of their generation, instead of the unhindered growth of antibiotic-resistant strains. The result is that the resistance to AAT is possible but on a different scale than the dramatic race observed for antibiotics. (Cozens et al., 2012) Furthermore, the prospects of AAT will undoubtedly benefit from the lessons learned from antibiotics, such as the need to limit over-prescription and encourage combination therapies, among others.
Indeed, combination therapies may be instrumental in curbing otherwise unsurmountable MDR pathogens. Recently, modern computational tools became available to model and predict outcomes of combination therapies on simple disease models. Encouraging results showed that antibiotics and anti-adhesives combine synergistically, generating better outcomes than the isolated treatments. Furthermore, the study optimized the treatment to arrive at a predicted ‘best-case’ outcome, in which the minimum antibiotic dose was lower, reducing the chances of resistance to develop.(Roberts et al., 2019) Thus, it would seem that AAT coupled with the gathered knowledge and the newest technologies can turn the tide in the fight against pathogens.
Among the different AAT approaches against infections, some highlights include the disruption of the biosynthesis of adhesion factors of either pathogen or host, the use of antibodies targeting adhesion factors, the immunization of patients against the adhesion and the competition against binding epitopes by tailored therapeutic agents. (Krachler et al., 2013) We will develop this last example: the design of AAT agents intended to mimic and compete against epitopes that are usually targeted during the adhesion process in the context of early infection.
As mentioned earlier, adhesion is a staple of infection, meaning that adhesion machinery has evolved throughout time and become increasingly effective and varied. This machinery has also gained specificity in its variety: many different virulence factors specifically target their corresponding epitopes in the host/pathogen interface. Consequently, an understanding of these virulence factors, their targets and the host/pathogen interface is necessary to attempt AAT. One key element of this same interface is the so-called glycocalyx: a carbohydrate-populated matrix that encapsulates different cells, including epithelial and bacterial cells.