Four steps may be distinguished in lignin formation :
1/ biosynthesis of monolignols ;
2/ transport of monolignols to the plant cell wall ;
3/ enzymatic formation of monolignol radicals ;
4/ coupling of the radical monomers into the growing lignin polymer. In spite of progress in the understanding of lignin metabolism, parts of those are still controversial.
Monolignol biosynthesis
Monolignol biosynthesis derives from the general shikimate pathway leading to the aromatic aminoacid phenylalanine in plants and other organisms but animals. Monolignols are synthesized through the general phenylpropanoid pathway to produce hydroxycinnamoyl-CoA esters (Fig. 3). In this process, phenylalanine is deaminated by phenylalanine ammonia lyase (PAL) (Higuchi 1990, followed by a series of successive hydroxylation reactions (catalyzed by hydroxylases ) of the aromatic ring, phenolic O-methylation (catalyzed by methyl transferases) followed by reduction (catalyzed by dehydrogenases) of the side-chain carboxyl to an alcohol group Higuchi 1990Dixon 2001 Boerjan et al., 2003 Vanholme et al., 2010. At least 10 enzymes are involved in this cascade of reaction : 4-hydroxylase (C4H), 4-coumarate CoA ligase (4CL) ; cinnamate hydroxycinnamoyl CoA:shikimate hydroxycinnamoyl transferase (HCT), p-coumaroyl shikimate 3-0-hydroxylase (C3H), caffeoyl CoA O-methyltransferase (CCoAOMT), ferulate 5- hydroxylase (F5H), caffeic acid O-methyltransferase (COMT), cinnamoyl CoA reductase (CCR) and cinnamyl alcohol dehydrogenase (CAD) Bonawitz and Chapple 2010Zhong, Ye 2015.
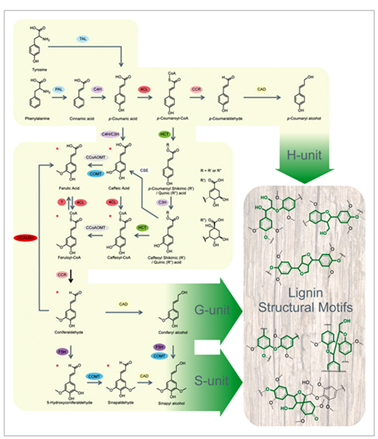
Monolignols transport to the plant cell wall
The next step after monolignols biosynthesis is their translocation from cytoplasm to the site of their polymerization in the cell wall. The process by which lignin precursors are transported across membrane to the apoplast then oxidatively polymerized in the cell wall form remains a subject of considerable debate Barros et al., 2015 Pandey et al., 2015. It is suggested that monolignols could be delivered in different ways at different cellular differentiation stages Sibout, Hofte, 2012. As underscored by Tsuyama et al. (2013 “Several transport mechanisms of lignin precursors across the plasma membrane can be hypothesized and classified into four patterns : passive diffusion, active primary transport, active secondary transport, and vesicle transport by the endomembrane system” (Fig. 4). The hypothesis of monolignols transport by simple diffusion has been suggested Boija and Johansson, 2006. Lignin precursors may be transported from the cytosol to the cell wall across the plasma membrane suggesting the involvement of a common endomembrane-associated proton/coniferin antiport mechanism in the lignifying tissues of woody plants, both in angiosperms and gymnosperms.
Monolignols are also suggested to be released from glucosides through ß-glucosidases. Recent studies have identified specific membrane protein transporters involved in active transport through transporters of the ATP-binding cassette (ABC) family, which mediates the passage of the monolignol p-coumaryl alcohol across the plasma membrane of vascular tissues at early stages Sibout, Hofte, 2012.
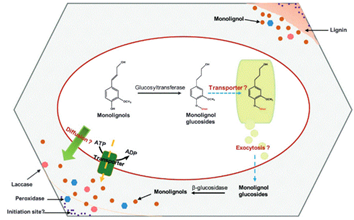
Radical formation and polymerization
Polymerization of monolignols into lignin macromolecules is a phenomenon taking place in muro, once they have been transported through the plasma membrane. The first step is their dehydrogenation via one-electron oxidation of phenols at the O4 position by oxidative enzymes to yield phenoxy radicals. Oxidation via a redox shuttle has also been suggested Ros-Barcelo et al., 2006. The mesomeric forms of the phenoxy radicals are resonance-stabilized due to delocalization of the unpaired electron in the conjugated system Freudenberg, 1959Higuchi, 1985 Boerjan et al., 2003 Zhang et al., 2012. It has long been thought that the catalysts responsible for the oxidation of monolignols were laccases and/or peroxidases. Peroxides (EC 1.11.1) are a superfamily of heme-containing enzymes that use hydrogen peroxide (H2O2) as the electron acceptor to oxidize their substrate, whereas laccases (EC 1.10.3.2) are copper-containing oxidase (p-diphenol:O2 oxidoreductases) enzymes that act on phenols and use dioxygen (O2).
The fate of the resonance-stabilized phenoxy radicals is to couple with each other or with a growing lignin polymer. The cross-coupling of phenoxy radicals gives rise to variously linked interunits connected through numerous linkages such as C-O-C (β-O-4, α-O-4, 4-O-5 etc.) and C-C (β-5, β-1, β-β, etc.). The most frequent β-aryl ether (8–O–4) units, the so-called non-condensed unit, and to the so-called condensed β–β (8–8) and β–5 (8–5) units. And less frequently occurring, the 5–5- and 5–O–4-linked units. The α-O-4, 5-O-4 and 8-membered ring dibenzodioxocin linkages result in branching in the chains.
Coupling of the radical monomers and polymerization
For a long time the polymerization of the radical monomers was thought to be a random process entirely dependent of the cross-coupling of the free radicals together and with a growing lignin polymer Freudenberg, 1959 Higuchi, 1990. Currently, there are two opposing theories about the polymerization and molecular assembly of lignin Hatfield, Vermerris, 2001 Önnerud et al., 2002 Ralph et al., 2004. An alternative to the random coupling of the phenoxy radicals model suggests that a non-enzymatic protein called the dirigent protein (DiP) guides the coupling of the radicals by a template mechanism that controls the polymerization of lignin Davin, Lewis, 2000. As in the case of lignan dimers Lewis, Davin, 1998, the stereospecific control of the DiP would result in optically active products. According to Ralph and co-workers Ralph et al., 2004, the template theory does not fit with the racemic nature of cell wall lignins, whereas the model of chemically-controlled combinatorial coupling reactions is more in line with the actual analytical results. In this random theory, lignin polymerization is not totally uncontrolled and several factors are interfering, such as the rate of diffusion of the monolignols, the concentration of radicals or the supply of the peroxidase cofactor, H2O2. The influence of the polysaccharide environment of the cell wall upon random polymerization was pointed out by computational modeling (Houtman, Atalla, 1995). The β-O-4 units may carry side chain exhibiting (R,R/S,S) or (R,S/S,R) relative stereochemistry Njiojob et al., 2016.
The result itself of the polymerization is debated in terms of the molecular mass of lignin. Considerable discrepancies are observed in the number average molecular weight (Mn), weight average molecular weight (Mw), and polydispersity, reported for lignin depending on the biomass source, pre-treatment conditions, and isolation procedure, as well as the measurement technique employed, size-exclusion chromatography and MALDI-TOF giving the best results Baumberger et al., 2007 Tolbert et al., 2014.