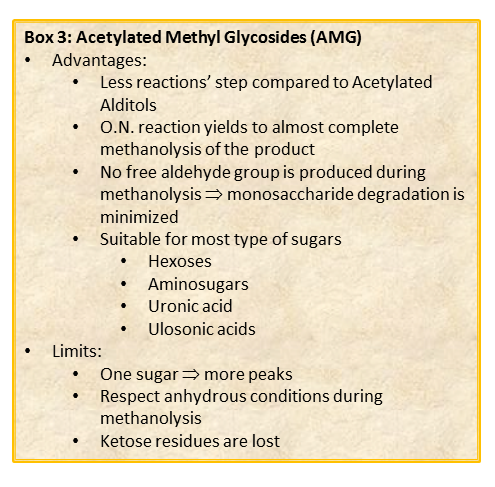
The chemical approach to produce these derivatives follows the scheme shown below
shown, and experimental details can be found elsewhere [link to “Further Readings”].
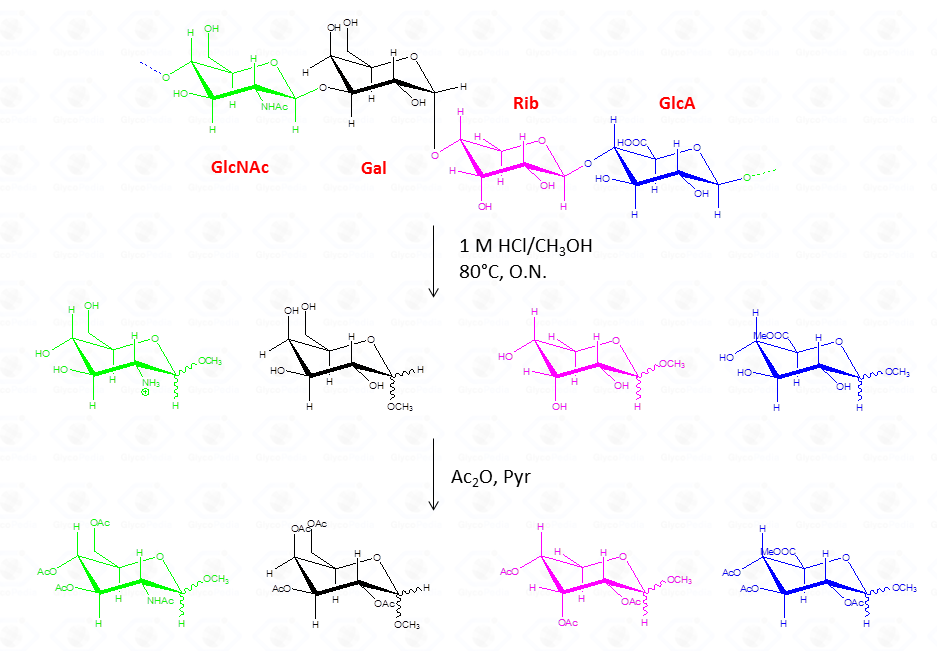
Whereas this procedure is suitable for the detection of almost all type of monosaccharides (neutral or basic aldoses, uronic and ulosonic acids), it fails in the detection of ketoses, as fructose. This approach differs from that of the acetylated alditols for many aspects because monosaccharides never expose their reducing end so that the parasite reactions of the aldehyde group do not occur. All this translates in an increase in the recovery yield of each component.
By this reaction mechanism each sugar produces a mixture of different glycosides, which differ for the configuration at the anomeric center (α and β ) as well as for the ring size (pyranic or furanic).
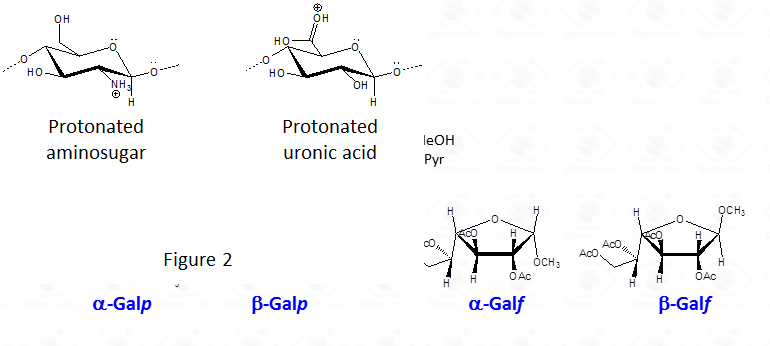
In the case of uronic acids intramolecular lactones may be produced.
With regard to the analysis of EI-MS spectra, is that, in common to acetylated alditols, different isomers have the same fragmentation pattern and it is not possible to discriminate among them on the basis of the spectrum.
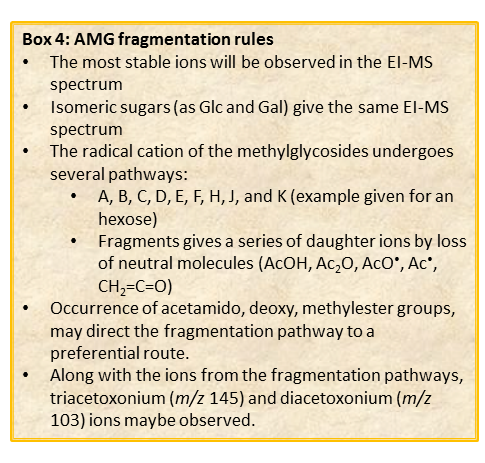
Different isomers must be discriminated by their retention time, which in turn depends on the type of GC-column used as well as on the experimental set up of the chromatographic apparatus of the instrument.
In general, the spectra from peracetylated methylglycosides do not contain the signal of the molecular ion. It can be indirectly deduced either by the occurrence of the primary ion formed (loss of acetoxyl radical (m/z 59)) or by the weak signals of two different oxonium ions which occur throughout the A and E pathways.
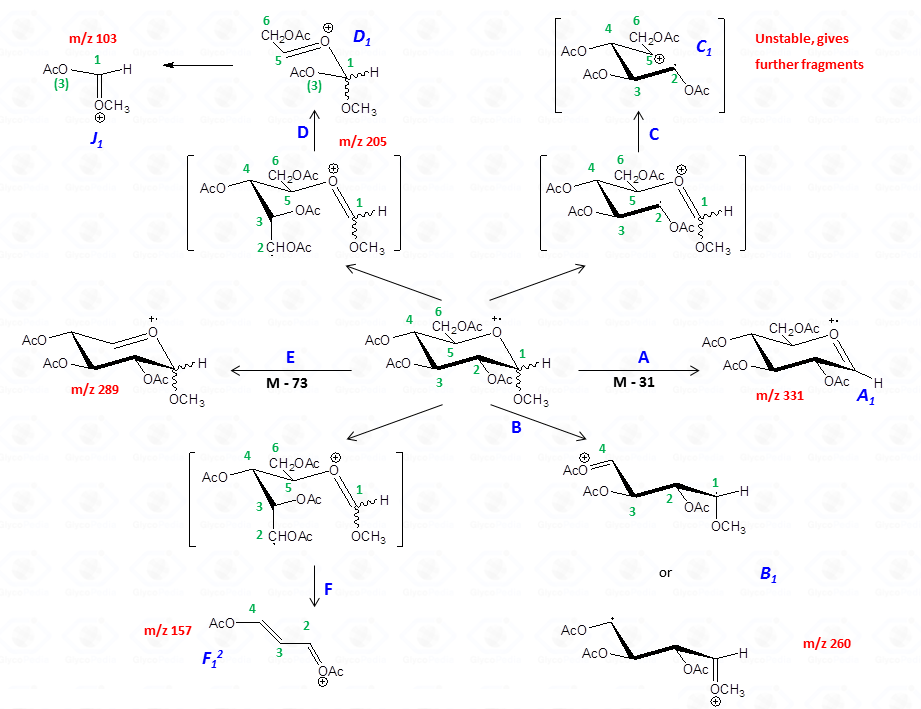
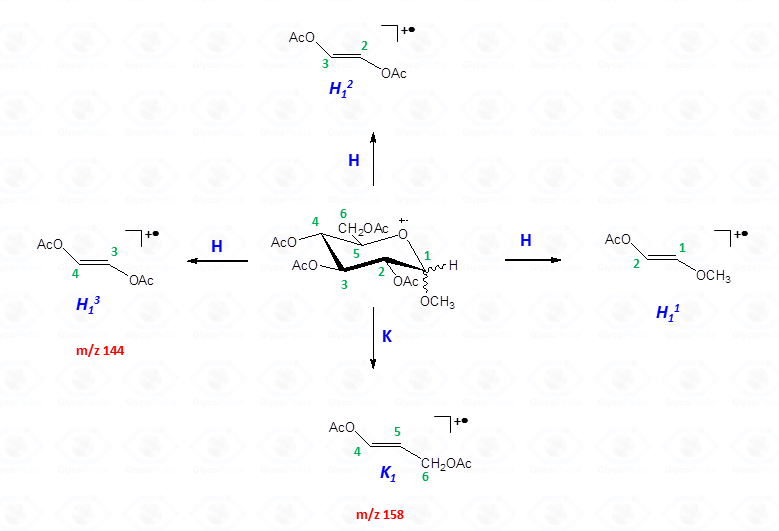
These fragments can further loose neutral moiecules, such as acetic acid (CH3COOH, m/z 60), acetic anhydride (Ac2O, m/z 102) or chetene (CH2CO, m/z 42)
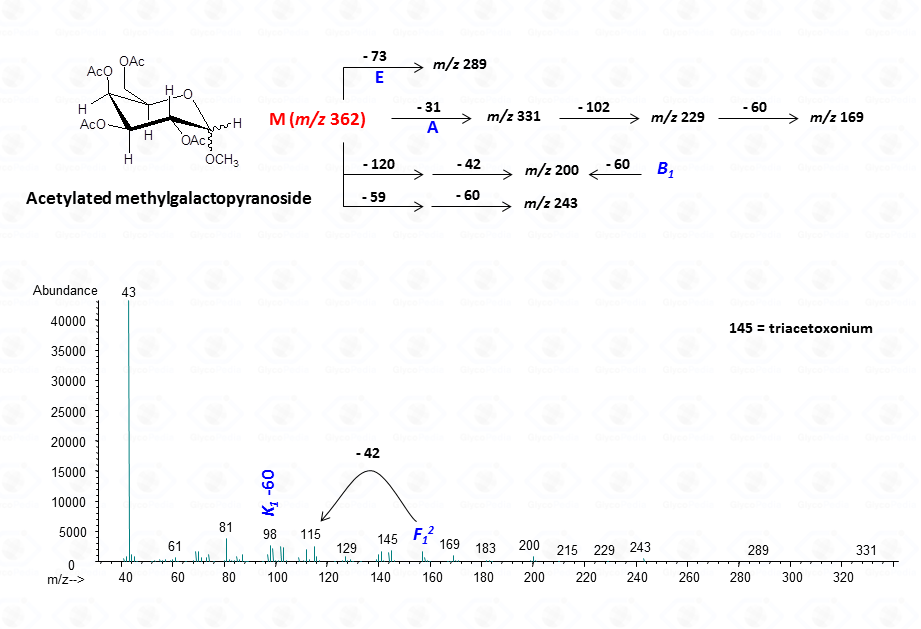
The other fragmentation pathways (see Figs 12a and 12b) yield signals in the low mass range of the spectrum that are less useful to deduce the structure of the residue.
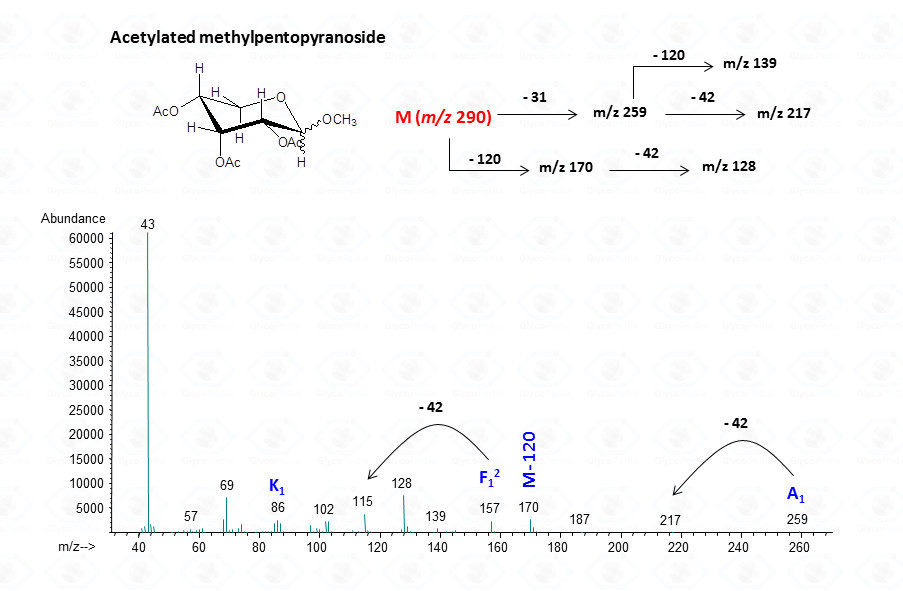
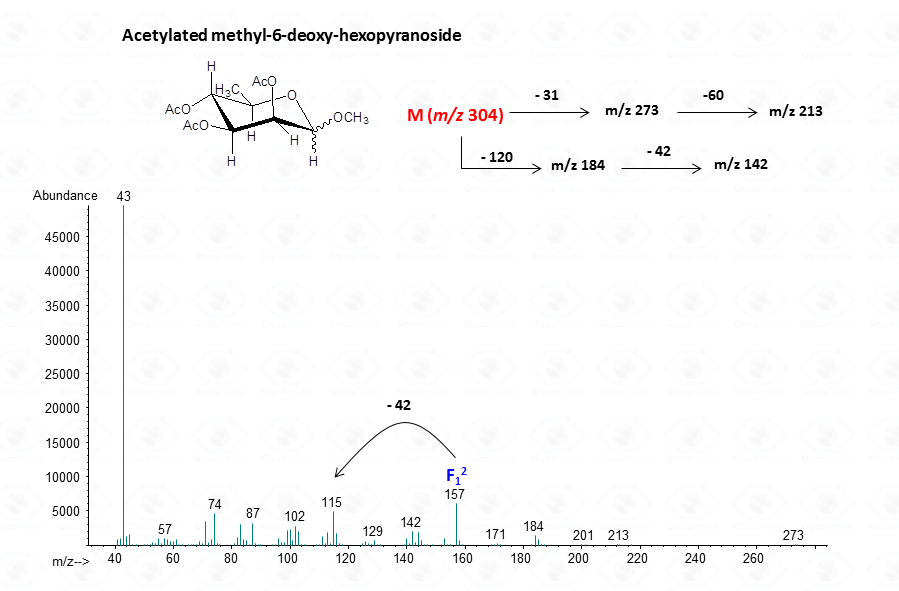
The acetylated methyl glycosides method allows detection of acidid monosaccharides. Indeed, uronic acids can be identified from their distinctive fragmentation pattern.
For the pyranose form
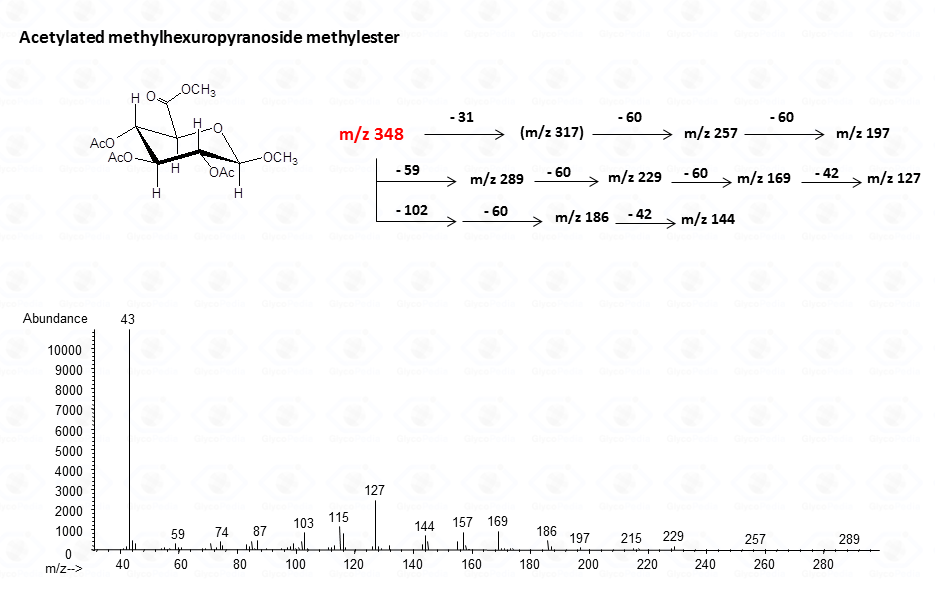
For the intramolecular lactone
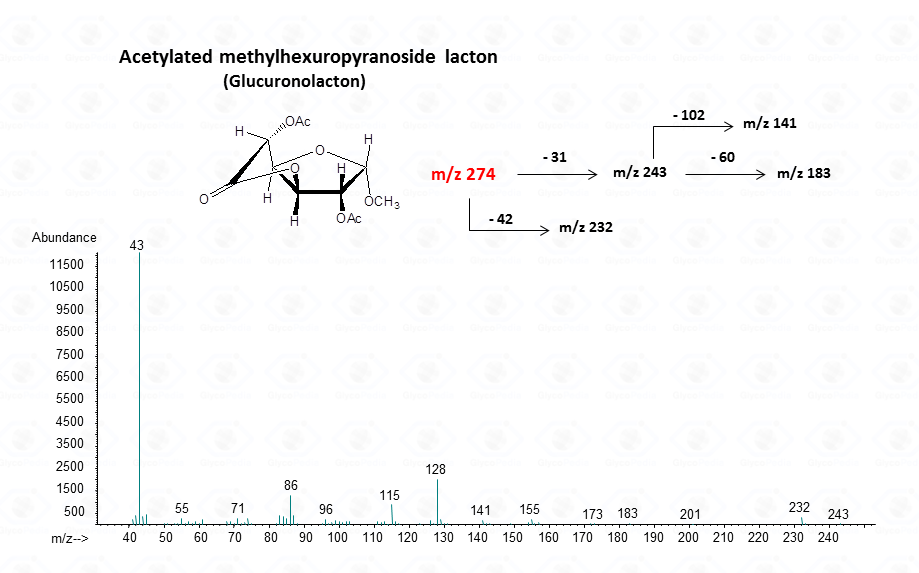
For ulosonic acids, as Kdo
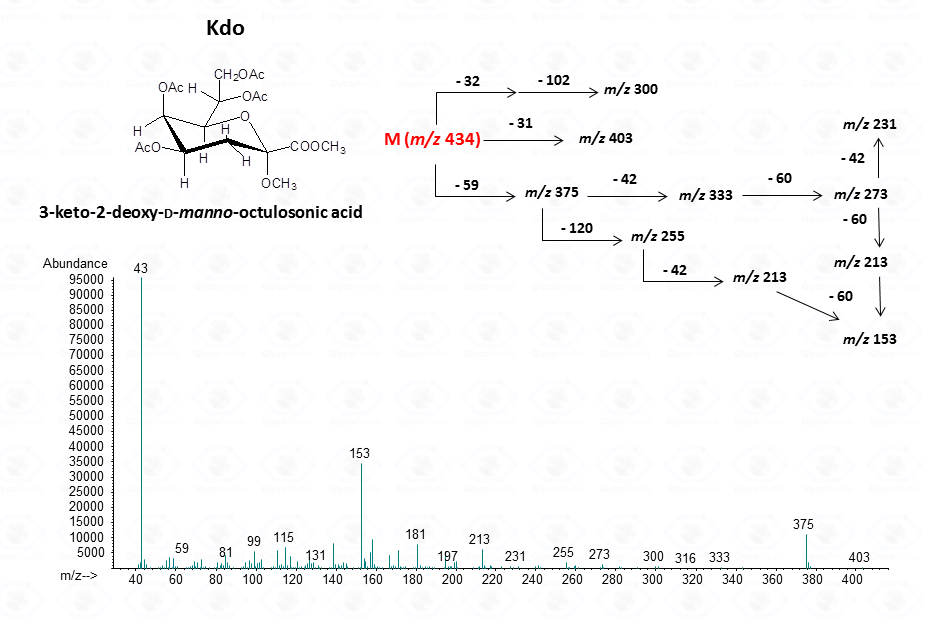
For sialic acid,
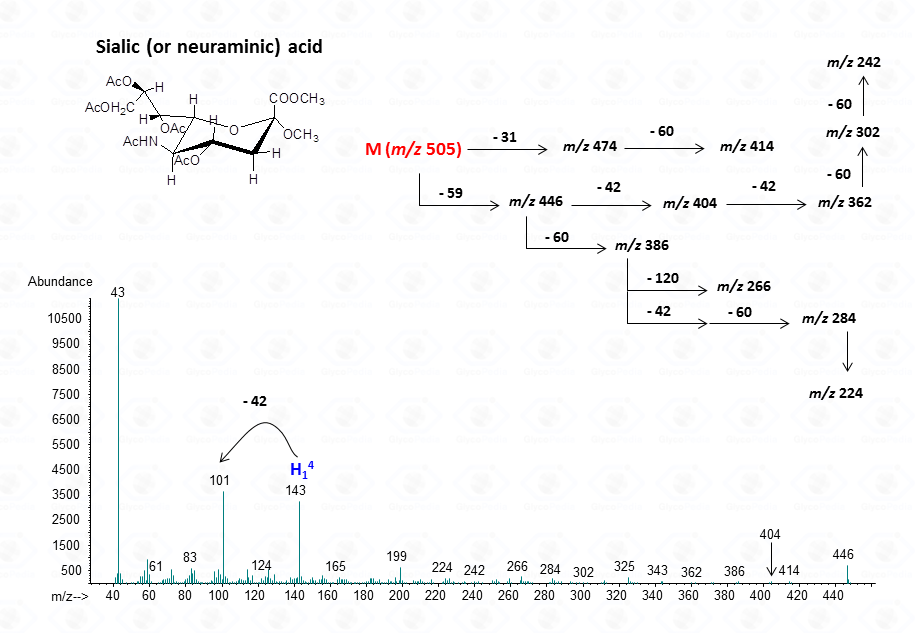
For legionaminic acid)
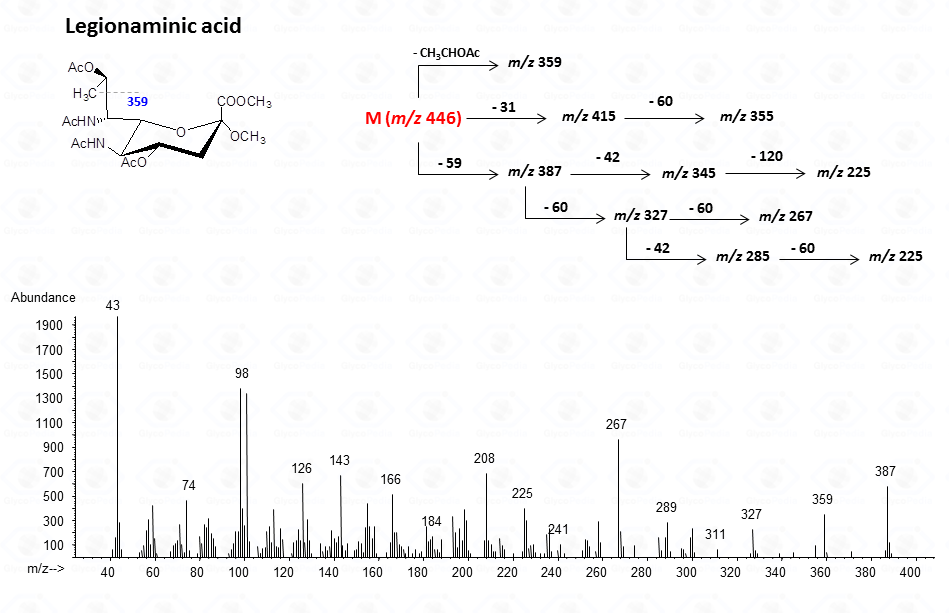
For aminouronic acids
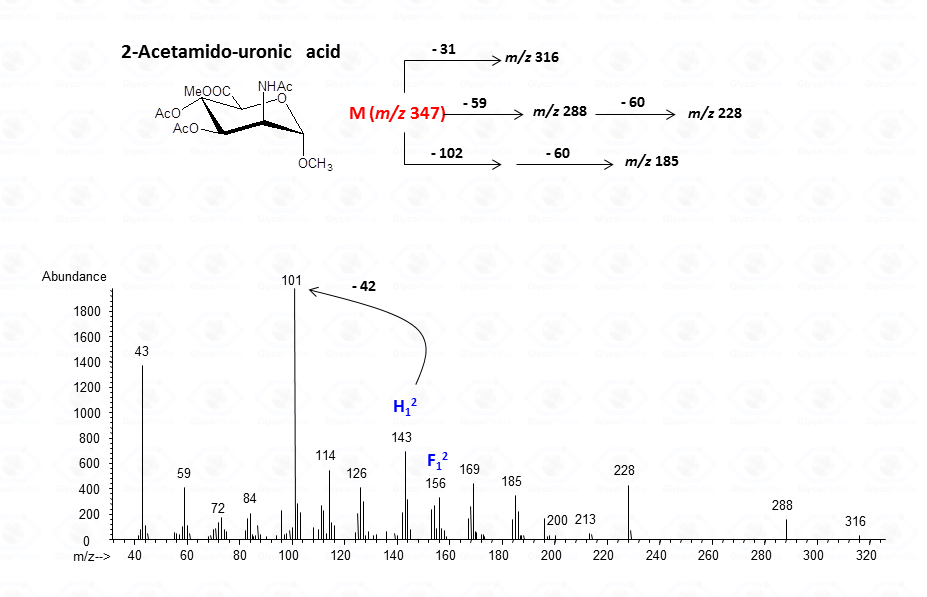
The lost from the molecular ion of the carboxymethyl group (derived from the acidic function) gives always a rather intense signal at high molecular mass range and this peak is highly informative of the composition of the compound. Clearly, the Acetylated Methyl Glycosides method finds its application also to aminosugars, as simple hexosamines (glucosamine in Fig. 22)
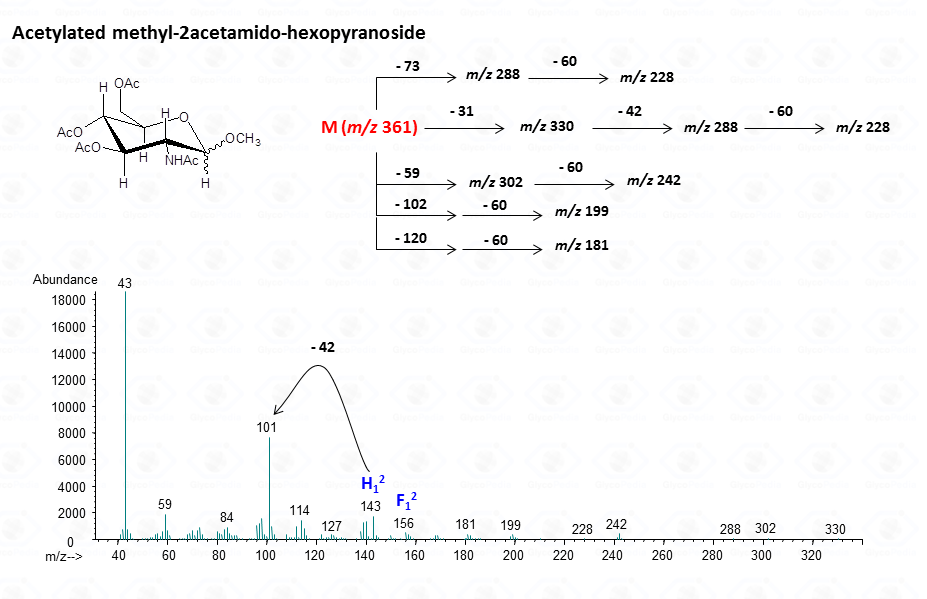
or to more complex residues, as the above mentioned aminuronic acids (Fig. 21), or muramic acid.
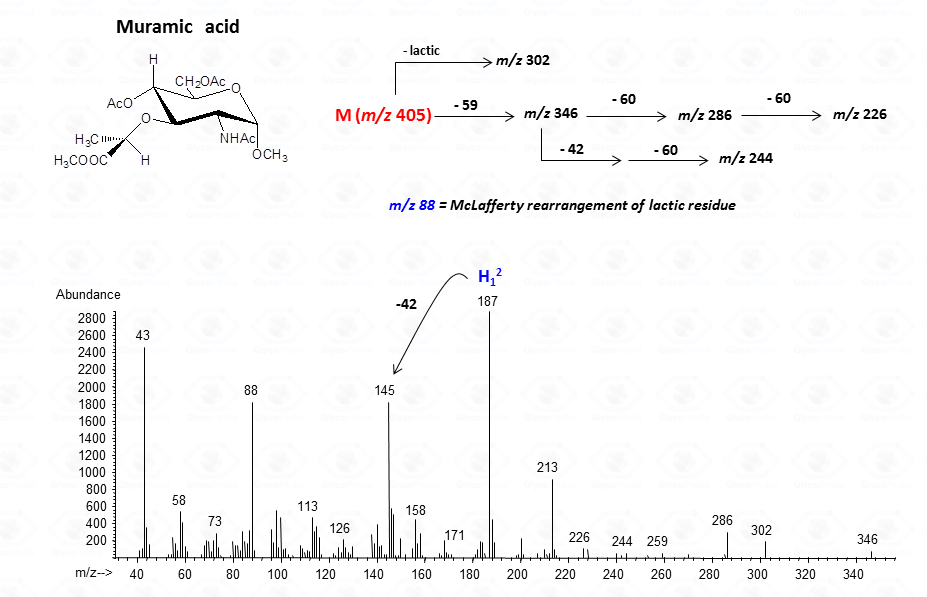
In few cases, this methodology can be used to discriminate among different sugar isomers, as the three isomeric 6-deoxy-N-Acetyl-hexosamines for which the spectra display small but nevertheless marked differences.
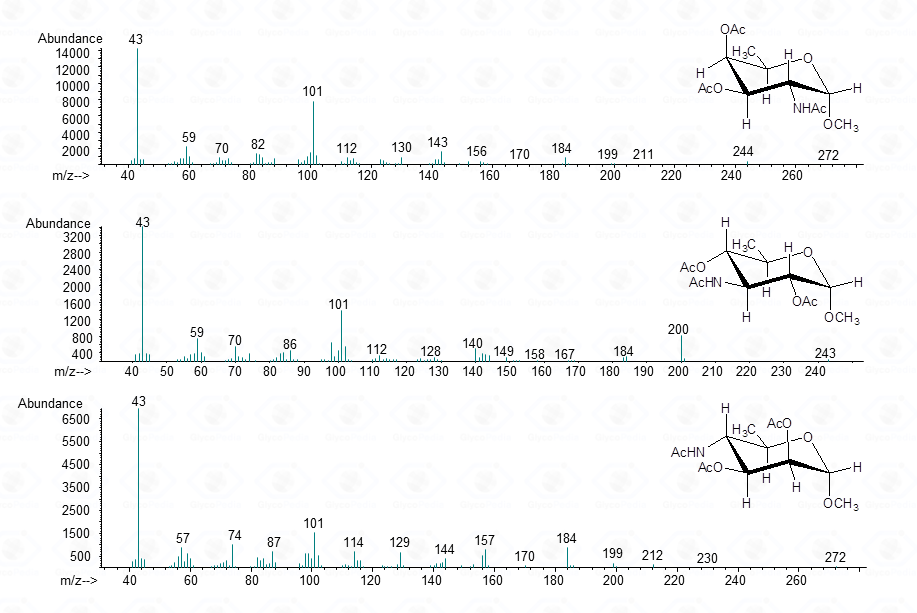
Such a differentiation is possible at the condition that the spectra of all the possible isomers are present in the library.
EI-MS fragmentation spectra contain all the information necessary for the identification of the monosaccharide components. Usually, the most informative peaks are those occurring at high mass ranges. In some cases, few diagnostic ions can be found in the low molecular range of the spectrum. This is exemplified by the comparison of the spectra obtained for a hexose (Fig. 13), a pentose (Fig. 14) and a 6-deoxyhexose (Fig. 15). The acetylated methyl glycosides of the hexose presents m/z 200 as diagnostic peak, whereas m/z 128 and 170 occur for the pentose and m/z 142 and 184 for 6-deoxyhexose, respectively. Clearly, during the process of monosaccharide identification, it is always safe to cross-check the results gathered from the fragmentation pattern data with other information, as those derived from the retention time of the suspected monosaccharide unit.
Acetylated methyl glycosides derivatives are stable standards. Once prepared, they can be stored and used for several years.