Selectin Ligands – Versatile Carbohydrate Epitopes.
Selectins
Selectins are carbohydrate-binding proteins that mediate reversible interactions with glycoconjugates enabling tethering and rolling of the leukocytes along the endothelium, a prerequisite for subsequent passage across the endothelial wall. (Zarbock et al., 2011 – Sperandio et al., 2009). E-selectin is expressed mainly on endothelial cells and P-selectin that can be rapidly displayed on platelets and endothelial cells upon stimulation are the most important selectins for this process (Kannagi et al., 2002 – Ley Tedder, 1995). L-selectins are expressed at the surface of many leukocytes, with capacity to induce tethering of blood cells to each other. L-selectins are also important in a variety of important selectin-dependent activities of circulating leukocytes, e.g. homing to peripheral lymph nodes(Kannagi et al., 2011 – Kawashima Fukuda, 2012). E- and P-selectins are expressed at the endothelial wall only after inflammatory stimulation (Bevilacqua et al., 1989). Thus, upon activation by mediators of inflammation including histamines, Tumour Necrosis Factor α (TNF- α) and lipopolysaccharide (LPS) resting endothelial cells can rapidly mobilize P-selectin to the cell surface from secretory granules Ley et al., 1995. Expression of E-selectin on resting endothelial cells also has to be activated by stimulation with TNF-α, LPS, interleukin-1 or other pro-inflammatory factors (Sperandio et al., 2003). Both E- and P-selectin can support recruitment of appropriately “authorized” T cells, monocytes, dendritic cells and neutrophils to the stimulated endothelium (Leon & Ardavin, 2008 – Austrup et al., 1997 – Bonder et al., 2005 – Robert et al., 1999 – McEver, 2002), priming their transmigration over the endothelium.
Selectin Ligands – Versatile Carbohydrate Epitopes
The main ligands for selectin binding expressed on activated leukocytes are carbohydrate epitopes, glycans, belonging to the Lewis family of glycoepitopes (Fig. 3)(Varki, 1994).
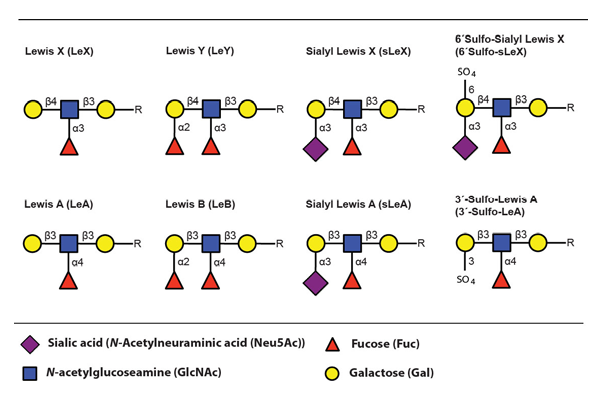
The sialyl Lewis X (sLeX) glycoepitope and structural relatives, i.e. sulphated variants, are the most important selectin ligands (Ohmori et al., 2006Wagers et al.,1997), but hereafter mainly sLeX will be considered for reasons of brevity. Like many other glycoepitopes sLeX can be associated with several types of glycoconjugates, including glycolipids and glycans of surface glycoproteins. There are two major classes of glycans associated with viral as well as host cell membrane proteins, designated N- or O-linked glycans owing to the nature of the linkage between the innermost glycan monosaccharide and the polypeptide backbone (Fig. 4)(Varki, 2009).
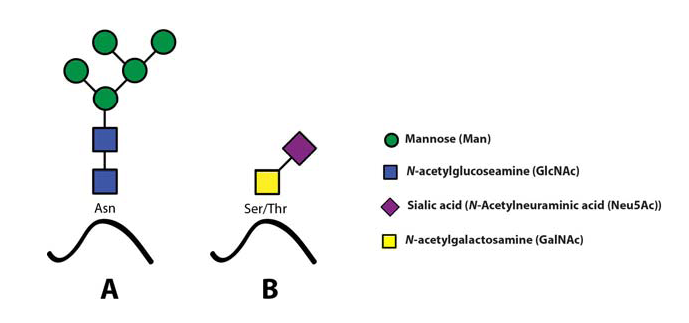
Both these two classes may express sLeXZarbock et al., 2011, but owing to its special relevance for our work, the present review will focus on sLeX as a constituent of O-linked glycoprotein glycans.
Glycoproteins that harbour selectin ligands
Although more than 50% of the human proteome represents proteins that carry glyco-modifications (Apweiler et al., 1999), only a handful of proteins (Table 2) are known to present selectin ligands in a way that promotes leukocyte transmigration (Garcia-Vallejo et al., 2008 – Berg et al., 1991 – Maly et al., 1996).
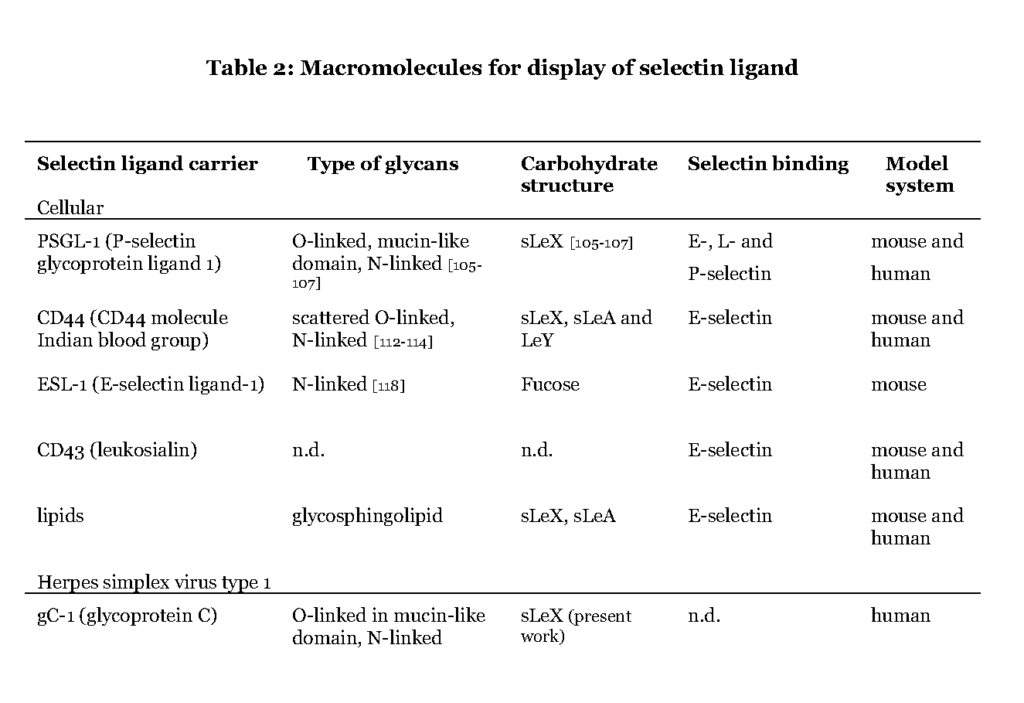
It is evident that the ability to express the particular carbohydrate epitopes (sLeX or related structures) as constituents of O-linked glycans is a common feature of these glycoproteins (Table 2). For one such protein, P-selectin glycoprotein ligand 1 (PSGL-1), the precise location of the relevant sLeX-carrying O-glycan has been determined (Wilkins, et al., 1996 – Afshar-Kharghan et al., 2001 – Moore et al., 1995).
Some of the glycoproteins presenting functional selectin receptors contain special mucin-like domains, allowing expression of multiple O-linked glycans along a short peptide stretch (Table 3 ; detailed below).
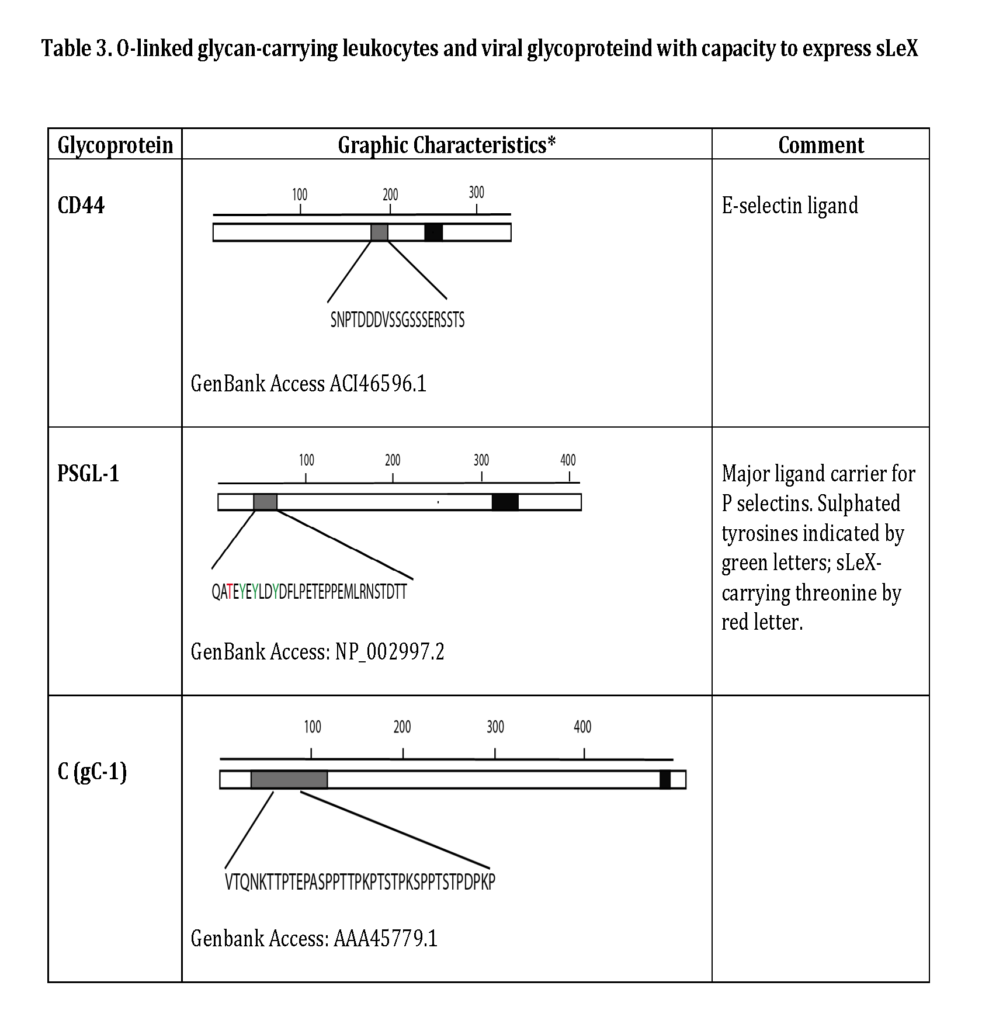
The selectin ligands bind to different selectins depending on the macromolecule it is displayed on, i.e. PSGL-1 associated sLeX facilitates binding to all selectins while CD44 carrying sLeX only mediates E-selectin ligand interaction (Table 2 and 3). Hence, the sLeX structure is essential for selectin binding but additional factors also regulate the interaction.
Biosynthesis of selectin ligands associated with O-linked glycans
Posttranslational glycosylation of proteins or sphingolipids requires the synchronous action of roughly 200 enzymes, glycosyltransferases, to a large extent located in the golgi compartment where they catalyse the transfer of activated sugar nucleotide donors to glycoconjugate acceptors, and approximately ten glycosyltransferases are needed for sLeX synthesis (Sperandio, 2006 – Gill et al., 2011 – Varki et al., 2009). The glycosyltransferases have three specificities (i) sugar specificity, i.e. the type of monosaccharide it can transfer, (ii) acceptor specificity and (iii) linkage specificity (Fig 5) (Roseman, 1970). The “one enzyme – one linkage” hypothesis postulated that each glycosyltransferase only generates one type of carbohydrate structure is an oversimplification but can help us understand the basics of glycan biosynthesis (Roseman, 1970).
The most important steps in the synthesis of an O-linked glycan carrying the sLeX epitope is depicted in Fig 6. Although single O-linked glycans may be scattered along the peptide sequence, several glycoproteins with peptide stretches enriched in Ser, Thr and proline (Pro) residues, referred to as mucin-like proteins, may contain multiple, clustered O-linked glycans. Thus, while the clustered Ser and Thr units serve as glycan carriers, the Pro residues enable access to the Ser and Thr units by “bending” the polypeptide backbone in an appropriate manner for the O-glycosylation machinery (Hanisch, 2001 – Wilson et al., 1991). Table 3 presents the mucin domain peptide sequences of two important selectin ligand carriers of glycoprotein nature.
After the first O-linked GalNAc unit is connected to the peptide, the remainder of the O-linked glycan is assembled by the concerted and coordinated actions of sequentially acting glycosyltransferases, each adding a unique monosaccharide in a unique position to the growing glycan (Lowe & Marth, 2003). The mode of action and specificities of these glycosyltransferases are illustrated in Fig. 6. Important for the regulation of sLeX synthesis is that essentially all of the glycosyltransferases operating in O-linked sLeX synthesis, except for the last one, are constitutively expressed (de Vries et al., 2001 – Lofling, & Holgersson, 2009 – Barthel et al., 2009 – Tenno et al., 2007), resulting in an accumulation of the direct precursor to sLeX, “the sialylated core 2-precursor” (Fig 6). In contrast, the genes encoding fucosyltransferases carrying out the last step are normally switched off (de Vries et al., 2001 – Lofling & Holgersson, 2009). Therefore, the rate limiting step and hence also the switch mechanism for inducing sLeX formation is activating one or more of these critical fucosyltransferase genes.
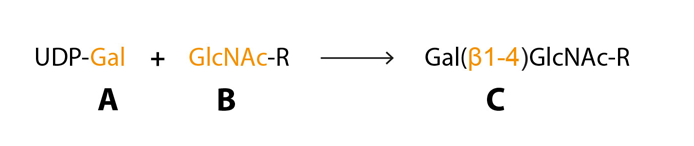
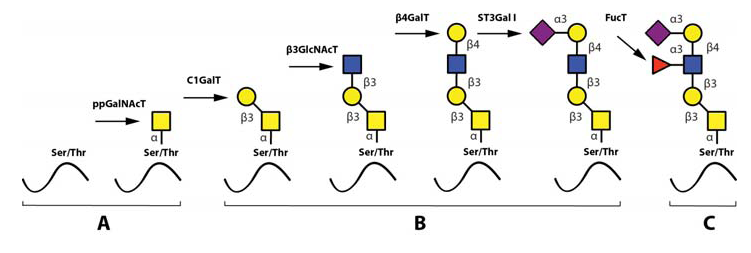
Regulating of the rate-limiting step for sLeX synthesis
Initiation of sLeX formation means activation of the very last rate-limiting addition of fucose to the sialylated core 2-precursor. The human genome contains 13 genes, each encoding an enzyme, fucosyltransferases (FucT), with the capacity to add fucose residues to glycoprotein glycans or peptides (Table 4) (de Vries et al., 2001 – Lofling & Holgersson, 2009 – Barthle et al., 2009 – Tenno et al., 2007 – Ma et al., 2006).
The nomenclature of genes and their products are as follows : The genes are enumerated FUT1-FUT13, whereas the corresponding gene products (enzymes) are designated FucT-I to FucT-XIII. Of these enzymes, only FucT-III, FucT-V, FucT-VI and FucT-VII (encoded by FUT3, 5, 6 and 7) are able to add fucose in the specific alpha 1,3 linkage to the sialylated type 2 precursors, which is a prerequisite to create sLeX (de Vries et al., 2001). The fine specificities of the FucT:s with capacity to generate the sLeX-characteristic alpha 1-3-fucosidic linkage varies from enzyme to enzyme (Table 4). For example FucT-V is a promiscuous enzyme, which accepts all four variants of sialylated or nonsialylated core 1 or core 2-precursors. Moreover, this enzyme can form alpha1-3 as well as alpha 1-4 linkages, should the appropriate precursor be available. Hence, this enzyme is able to synthesize not only sLeX but also Lewis X (LeX), Lewis Y (LeY), sialyl Lewis A (sLeA), Lewis A (LeA) and Lewis B (LeB), depending on the identity of particular precursors available in the tissue expressing FucT-V (Table 4). In contrast, fucosyltransferase VII (FucT-VII) is highly sLeX-specific since this enzyme is able to address only the sialylated core 2-precursor, preventing synthesis of any other structures of the Lewis family of glycoepitopes, provided that no other relevant fucosyltransferase-encoding genes are expressed. Thus, FucT-VII has a narrow specificity and can only synthesize sLe (de Vries et al., 2001 – Ma et al., 2006 – Kannagi, 2001). FUT4 and FUT7 encode the only fucosyltransferases expressed in leukocytes, determined so far, and both are important for proper selectin ligand display (Kawashima &Fukuda, 2011 – Sperandio, 2006 – Ma et al., 2006 – Kannagi, 2001). Loss of FUT4 and/or FUT7 functional gene products significantly affects E- L- and P-selectin dependent binding at least in mice (Maly et al., 1996 – Weninger et al., 2000 – Homeister et al., 2001 – Bengtson et al., 2001 – Bengtseon et al., 2002).
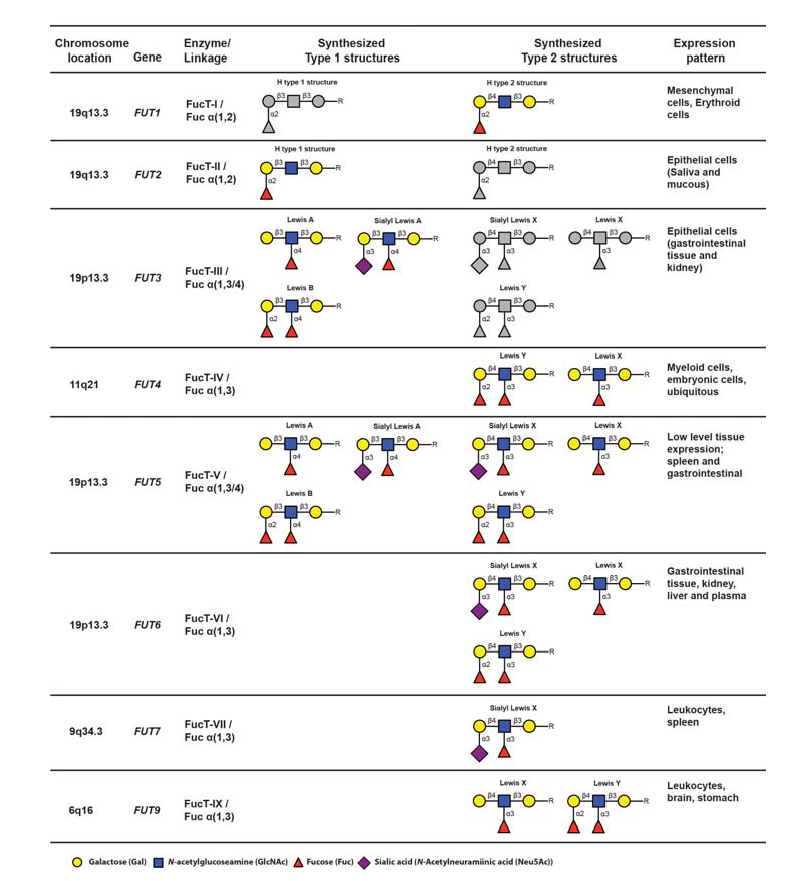
Normally an inflammatory stimuli of the leukocyte by interleukin 12 (IL-12) and transforming growth factor α1 (TGF-α1) activate expression of FUT7 and this functions as an on-switch for the synthesis of sLeX in resting leukocytes (Barry et al., 2003). Activation-dependent transcription factors, including T-cell-specific T-box transcription factor (T-bet) and CRE-binding protein (CREB)/activating transcription factor (ATF), induce FUT7 transcription upon the stimulation. This process is reversible in normal situations and loss of external stimuli results in down modulation of FUT7 expression and loss of sLeX (Kannagi et al., 2011 – Chen et al., 2006).
Related glycoepitopes of the Lewis family
The fucosyltransferases are capable of generating a large collection of carbohydrate epitopes related to sLeX, many of them differing only in linkage specificity between the monosaccharides (Fig 3 and Table 4). Of particular interest is the Lewis Y (LeY) epitope, which is dependent on expression of the H type 2 precursors by FucT-I (FUT1) for its synthesis. LeY can be found in CD34+ hematopoetic precursor cells but is absent in mature lymphocytes isolated from both the blood and from the tonsils (Cao et al., 2001). The LeY glycan can also be found in certain leukemic cell lines and abnormal expression is, like sLeX, highly associated with malignancy and strongly correlates to poor prognosis (Miyake et al., 1992 – Nakamori et al., 1993). Recently it was shown that LeY expressed on tumour cells mediate spread to the lung via interaction with Srfs proteins displayed on lung endothelial cells (Hatakeyama et al., 2009). This indicates that other carbohydrate structures related to sLeX can function as mediators of transmigration and thereby act as tags for leukocyte homing.
It is well established that the modified sLeX structure, 6-sulfo sialyl Lewis X (6´Sulfo-sLeX) (Fig. 3), is important for L-selectin mediated homing by lymphocytes to high endothelial venules (HEV) in peripheral lymph nodes (PLN) and it is also expressed by subsets of T lymphocytes destined for routine homing to the skin (Kanamori et al., 2002 – Kimura et al., 1999). Lymphocytes obtained from healthy individuals mainly express this sulphated derivate of sLeX, which they use for routine migration in and out of tissue through interaction with E- and P-selectin expressed at the lining of dermal blood vessels (Ohmori et al., 2006). This contrasts to the situation in patients with inflammatory disorders where activated T lymphocytes largely express the standard sLeX epitope (Munro et al., 1992).
Sialyl Lewis A (sLeA) is a Lewis structure that is similar to sLeX, differing only in the specific linkage between the distal galactose and its neighbouring GlcNAc (Fig. 3). This epitope is atypically expressed in tumours of various origins and contribute to the metastatic potential (Sakuma et al., 2012 – Yin et al., 2010 – Markine-Goriaynoff et al., 2004). It was recently shown that sLeA can be displayed on glycoprotein CD44, mediating polymorphonuclear leukocyte (PMN) transmigration over the intestinal epithelium (Brazil et al., 2013). Altogether this suggests that other Lewis structures can function as “address tags” during leukocyte homing, guiding the cells to specific areas of the body.
Molecular mechanisms behind tumour and viral hijacking of selectin functions
Various tumour cells can induce expression of selectin ligands, e.g. sLeX, by activating FUT-genes and this contributes to their potential for colonizing new tissue (Kannagi et al., 2004). It is generally not known how the activation of fucosyltransferase-encoding genes is accomplished in cancer cells, only that the ability to induce selectin ligands strongly correlates to metastatic capacity and poor prognosis. The retrovirus HTLV-1 triggers transformation of the infected lymphocyte, causing lymphoma. The virus induces FUT7 transcription, which leads expression of sLeX on the cell surface and this contributes to the skin infiltrating capacity of the transformed cells (Kanngi, 2001). The mechanism behind transcriptional activation of FUT7 is well described for HTLV-1 infected leukocytes. The virus-encoded protein Tax carries out transactivation of FUT7, via association with CREB, in HTLV-1 infected leukocytes (Hiraiwa et al., 2003), bypassing the need for Il-12 and TGF-1 stimulus in normal activation. One important difference compared with the normal situation is that Tax confers a constitutively activation of sLeX synthesis which is not reversible, leading to the strong tissue invasive nature of adult T cell leukemic cells (Hiraiwa et al., 1997Hiraiwa et al., 2003).
Cytomegalovirus (CMV) can activate expression from several fucosyltransferase genes including FUT1 upon infection in human embryonic lung fibroblasts (HELF), leading to expression of LeY as well as sLeX, albeit not simultaneously, on the surface of the infected cell (Nystrom et al.,2007). Also, varicella zoster virus (VZV) can induce sLeX in fibroblasts (Nystrom et al., 2007) and herpes simplex virus type 1 (HSV-1) can activate expression of FUT5 that encodes fucosyltransferase V (FucT-V) in fibroblasts (Nystrom et al., 2004).
It appears that different types of human herpesviruses can interfere with the cellular machinery for Lewis antigen synthesis, and that the different herpesviruses can induce diverse fucosyltransferases for this purpose. Only two mammalian viruses have been found to encode any own glycosyltransferase and none of these viruses infect humans (Markine-Goriaynoff et al., 2004), implying that any human viruses strategy to induce novel glycoepitopes must be based on viral modification of host-encoded glycosyltransferase gene expression.
Manipulation of selectin ligand synthesis by HSV-1
Recently the details of the mechanism by which herpesviruses can induce selectin ligands in the infected host cell were delineated using HSV-1 as a model system. Due to a short replicative cycle of HSV-1 it was possible to determine that execution of the early viral RNA transcription program is necessary for activation of relevant fucosyltransferases in fibroblasts (Nystrom et al., 2009). The finding that the cellular antiviral protein kinase R (PKR) is a vital component of the HSV-1 mediated induction of FUT3, FUT5 and FUT6 in fibroblasts further supports the notion that expression of viral RNA is necessary for HSV-1 mediated induction of sLeX (Norden et al., 2013).
The natural target cells for blood borne spread of herpesviruses are various types of leukocytes. Infection of T cells with HSV-1 indeed activates expression of relevant fucosyltransferases and results in a display of selectin ligands on the cell surface (Norden et al., 2013). However, the mechanism behind HSV-1 mediated activation of selectin ligands in leukocytes appear to be different from the situation in fibroblast, in as much the viral protein ICP0 is implicated in activation of FUT7 instead of strict RNA dependent activation. Also, in addition to FUT3, 5 and 6 the HSV-1 infection in T cells can lead to the induction of both FUT1 and FUT7 underscoring the complexity viral mediated manipulation of selectin ligand synthesis.
As mentioned before, there are only a handful of human glycoproteins that harbour selectin ligands that are confirmed to bind selectins (Table 2). In the absence of host glycoproteins that are able to display selectin ligands HSV-1 encodes viral glycoprotein C (gC-1) that has the capacity to harbour large O-linked glycans in a mucin-like domain. It is suggested that this domain contains O-linked glycans holding distal sLeX (Norden et al., 2013). However, it still remains to be determined if sLeX displayed on gC-1 can facilitate binding to any of the human selectins and if this have any significance for spread of HSV-1 in the host. The mechanism behind HSV-1 induction of selectin ligands in fibroblasts and leukocytes is summarized in Fig. 7.
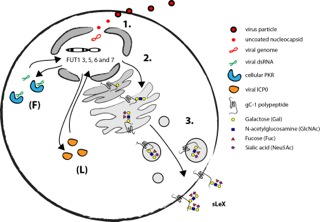